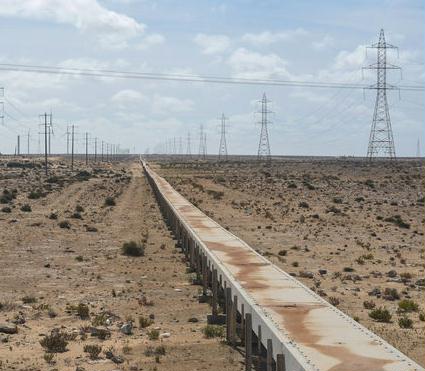
Source: The world’s longest conveyor belt system of 61 miles to convey phosphate ore to the sea (atlasobscura.com)
Preface. Phosphate is absolutely essential for both plants and animals. It’s estimated that Morocco has of 75-85% of phosphate reserves that might last for 300-400 years. Or peak in 25 years. Walan (2014) has estimates of researchers who’ve predicted peak phosphorus. But peak production will come early if Morocco and other key places experience supply chain failures, wars, economic depressions, lack of water, difficulty removing cadmium which is very toxic to plants.
China is the world’s largest producer of phosphate rock (48% of the world’s supply in 2013). It also uses a large amount of phosphorus to sustain its growing population. But China’s reserves of phosphorus, a key element for growing food, could be exhausted within the next 35 years if the country maintains its current production rate (Liu 2016).
Inevitably, the combination of rising cost and declining oil will force phosphate production to peak and then decline, even in Morocco (Bardi 2009).
If no action is taken decades before the anticipated peak, a hard-landing response to peak phosphorus is likely to result in a situation of (Cordell 2011c):
• increased energy and raw material consumption
• increased production, processing and transport costs
• increased generation of waste and pollution
• further short-term price spikes
• long-term trend of increased mineral phosphate prices
• increased geopolitical tensions
• reduced farmer access to fertilizer markets
• reduced global crop yields
• increased global hunger
Phosphorus may be the 11th most common element on earth, but each of these factors shrinks the amount available by further and further amounts until only a tiny amount is available for consumption:
1) Sufficient concentration of P
2) Found and physically accessible
3) Economically, energetically, legally, and geopolitically feasible
4) Available for fertilizer minus substantial mine-to-field losses
5) Plant available (P in soil solution)
6) Available for food minus substantial field-to-food losses
7) Available for consumption (minus food waste)
Clearly wars, supply chain failures, energy shortages / crisis, depressions, and more will also limit production.
Peak Phosphorus in the news
2021. Humanity Is Flushing Away One of Life’s Essential Elements. We broke phosphorus. The Atlantic.
2020. Researchers quantify worldwide loss of phosphorus due to soil erosion for the first time. More than 50% of global phosphorus loss in agriculture is due to soil erosion. Africa, Eastern Europe & South America have the greatest phosphorus losses—with limited options for solving the problem because fertilizers are too expensive for most farmers. Also, Africa has too little green fodder and too little animal husbandry to replace mineral fertilizers with manure and slurry.
Jaere. 2020. ‘Peak phosphorus’ is upon us, and sewage is valuable muck. Phys.org
Mohr S.H., et al. 2013. Projections of future phosphorus production. Philica Article number 380.
Alice Friedemann www.energyskeptic.com Author of Life After Fossil Fuels: A Reality Check on Alternative Energy; When Trucks Stop Running: Energy and the Future of Transportation”, Barriers to Making Algal Biofuels, & “Crunch! Whole Grain Artisan Chips and Crackers”. Women in ecology Podcasts: WGBH, Jore, Planet: Critical, Crazy Town, Collapse Chronicles, Derrick Jensen, Practical Prepping, Kunstler 253 &278, Peak Prosperity, Index of best energyskeptic posts
***
Walan, P., et al. 2014. Phosphate rock production and depletion: Regional disaggregated modeling and global implications. Resources, Conservation and Recycling 93: 178-187.
It’s important to note that many of these assume production at current rates. But as human population continues to grow exponentially, the rate of extraction is likely to increase, not remain the same as the year of publication. The aim of this study is not to provide accurate predictions of future production of phosphate rock, and certainly not to foretell a date of a peak in production. What this study does attempt to show is that large global reserves of phosphate rock do not necessarily mean large annual production.
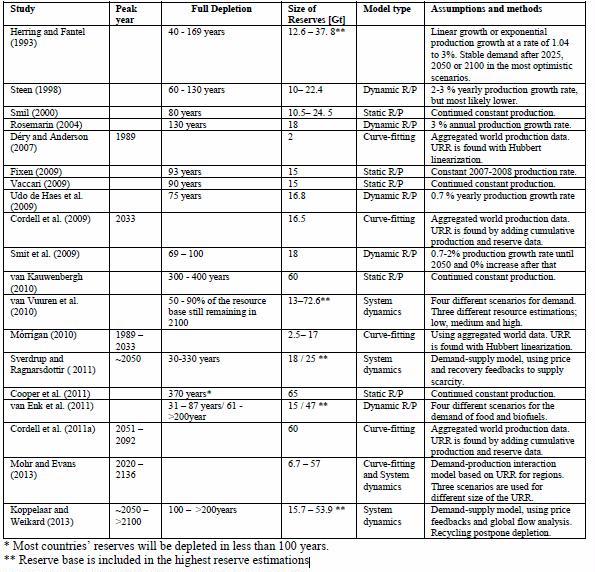
Table 1. Main features of previous studies on phosphate rock depletion and production.
* Most countries’ reserves will be depleted in less than 100 years.
** Reserve base is included in the highest reserve estimations
We conclude that the total estimated recoverable amounts of phosphate rock will likely not be the most important limiting factor for the global production in the near future, but what is commonly neglected is that the global supply could come to rely almost completely on one single country, namely Morocco. This means that potential bottlenecks and concerns about phosphate rock production need to be analyzed in the context of the individual countries, in particular Morocco. A main question for further research is if it is possible and even desirable, both for Morocco and the importing countries, that production in Morocco should increase as much as are implicitly indicated in some projections for future phosphate rock production. Also, even if the whole Moroccan current reserves estimate is extractable, it is still a risk that global production will experience a peak as a result of the declining production in China.
Future demand, as well as the quantities of phosphate rock available for extraction is highly uncertain, but what is absolutely clear is that the current depletion of phosphate rock cannot go on forever and a more sustainable use of the essential element should be desired for a multitude of reasons. Although the future of phosphate rock production appears uncertain, even the possibility of reaching a “peak phosphorus” calls for a timely transition to a more sustainable use of the resources, with more widespread reuse, recycling and higher efficiency in use of fertilizers as potential mitigation measures to depletion.
For mineral resources, such as phosphate rock, high-grade mineral ores exist in finite quantities and extraction will eventually lead to an exhaustion of the resources that are economic to extract, at the same time as demand for minerals will likely continue to rise (May et al., 2012). Easy extractable deposits of phosphate rock, with a high content of P2O5, are often used first, while low P2O5 content deposits results in more impurities and higher production costs (Van Kauwenbergh 2010). Some studies claim that global concentrations have been, and are currently, declining steadily (Schröder et al., 2009; Cordell et al., 2009; Vaccari 2011). Such a trend imply that the “easy deposits have already been exhausted and that future production would be forced to develop lower quality deposits with more associated costs and challenges (UNEP, 2011). This has been described as a vital mechanism behind the generation of production peaks (Bardi, 2009).
Phosphorus (P) is among the most abundant elements and is ranked as the 11th most common in the earth’s crust (Krauss et al., 1984), and the 13th most common in seawater (Smil 2000). However, it does not occur in its elemental form in nature due to high reactivity. About 95% of all crustal phosphorus is estimated to be bound in different forms of phosphate apatite minerals of which there are more than 200 known variants (Krauss et al., 1984). Phosphate rock deposits that are interesting for mining usually only occur under special conditions in some specific areas as a result of the phosphorus cycle (Filippelli 2011; Krauss et al., 1984). The main phosphate rock deposits are either sedimentary or igneous, each with different mineralogical, structural, and chemical properties (Van Enk et al., 2011). Marine sedimentary deposits account for 80% of the global phosphate rock production, with large producers such as China, Morocco, U.S. and Tunisia. Sedimentary phosphate rock ores commonly have a P2O5 content of around 30-35% (Krauss et al., 1984). Igneous deposits are low grade in comparison, with a P2O5 content of often less than 5%, but can be upgraded through beneficiation to 35-40% or even higher. While igneous sources contribute with 15-20% of current production, they constitute much smaller fractions of estimated resources. In contrast to sedimentary phosphate rocks, igneous deposits are generally free from pollutants such as radionuclides and heavy metals (Smit et al., 2009). Igneous deposits can mainly be found in countries like Russia, Brazil, and South Africa.
Data availability of reserves and resources of phosphate rock is often poor, in part as mining companies and fertilizer industry have limited interest in making detailed data publicly available, thus forcing analysts to rely of 2nd and 3rd hand information (Cordell 2011; Gilbert 2009). It is also sometimes argued that the phosphate rock deposits in countries like Morocco are not fully explored, since mining companies refrain from expensive exploration of potential reserves that are not expected to be put in production in the near term (Van Kauwenbergh 2010). It also appears like reserve data from China excludes smaller mines, implying that actual reserves might be larger than officially reported (Cordell et al., 2009). Another potential source of misconceptions is that reserve data is sometimes presented as phosphate rock ore and sometimes phosphate rock concentrate. Reserves specified in tons of phosphate rock are often assumed to be the same as the recoverable amount of phosphate rock concentrate, even if reserves often actually are phosphate rock ore that must be beneficiated in order to be sold, which normally requires a P2O5 content of 30% (Edixhoven et al., 2013).
The global resources are estimated by the USGS to be about 300 Gt of phosphate ore, out of which about 67 Gt are considered currently economically recoverable reserves (Jasinski, 2013a). According to Edixhoven et al. (2013), the USGS reserve data is routinely assumed to be listed as phosphate rock concentrate, while it appears that USGS often list reserves in terms of ore. Edixhoven et al. (2013) also claims that more than half of the phosphate rock concentrate in the resources consists of the reserves.
USGS estimates have remained constant for many countries despite continuous production, while other countries have made significant changes in reserves. The USGS reserve estimates from 2001 to 2013 is depicted in Figure 1. The most notable change can be seen from 2010 to 2011 when the estimated reserves in Morocco was multiplied many times, leading to Morocco now comprising 75% of the global reserves. However, it appears questionable whether all this is truly recoverable at current prices (Edixhoven et al., 2013; GPRI 2010).
Others argue that much more phosphorus is extractable, pointing to potentially massive quantities available in sea beds, continental shelves or even the sea water itself. Marine phosphate mining has never been done in large scale and the impact intensive dredging could have on the marine environment is unclear (Filippelli 2011). Consequently, environmental impacts have to be carefully examined before oceanic mining can be undertaken (Scholz 2013). Similar to many other elements, seawater is sometimes argued to be a more or less infinite phosphorus resource (IFA, 1998). The same argument is commonly presented for lithium, which is discussed by Vikström et al. (2013), who give an example of extracting all the lithium in 300,000 km2 of seawater, corresponding to the average discharge of the river Nile, would give roughly 20,000 tons of lithium per year. Since the sea water contains less P than Li, the same flow of seawater would contain the equivalent of about 11,000 tons per year, which is less than 0.1% of the current global phosphorus production. Considering the phosphorus would also need to be extracted from these immense amounts of water, with unavoidable losses, production from seawater at significant levels near the current or projected future demand must be considered very unlikely, even if this type of production were to come to occur at large scale.
Global phosphorus production
Phosphate rock mining originally started in South Carolina 1867 for manufacturing of phosphate fertilizers (Van Kauwenbergh 2010). In the early 20th century, new complex fertilizers were created that contained phosphoric acid, as well as ammonium nitrate and potassium chloride, commonly called NPK fertilizers (UNEP/UNIDO 2000). In the 1960s, new high yielding crop varieties were introduced, which contributed to large increases in crop production (Evenson 2003). What is sometimes neglected is that these varieties only give high yields if they can extract more nutrients from the soil and these new varieties, together with increased use of irrigation, also led to an increased use of fertilizer (IFA 1998). Phosphate rock production grew quickly and appeared to reach a peak in production in 1988 as a result of decreasing demand and production after the fall of the Soviet Union that coincided with an increased awareness of issues with eutrophication (Cordell et al., 2009; IFA, 2011, 1998).
In recent years, production has again started to rise, for reasons such as a growing global population, a sharp increase in the consumption of meat and dairy products, especially in growing economies such as China and India, as well as an increased biomass production for bioenergy purposes (Cordell et al., 2009). A more extensive description of phosphorus usage through history can be found in Ashley et al. (2011) or Cordell et al. (2009).
Phosphate rock is currently mined in more than 30 countries worldwide, but very few countries make up most of the total production (EcoSanRes 2008). The U.S. has dominated production historically, but appears to have peaked in production in 1980 at a production of 54.6 Mt of phosphate rock concentrate, and has fallen to almost half of this level (Déry 2007). The former Soviet Union countries used to be large producers, but have now fallen to around 5% of global production.
China is currently the largest producer in the world, accounting for 89 Mt or 43% of the total production of phosphate rock concentrates in 2012. China accounts for most of the sharp increase in production that has taken place since 2000.
After China, the largest producers are the U.S. and Morocco with roughly 14% of the global output each (Jasinski 2013a). Other important producers are primarily found in the Middle East North Africa (MENA) region with countries like Tunisia, Jordan, Egypt, Israel, Syria, Saudi Arabia, and Algeria. The MENA region, including Morocco, currently contributes with about one quarter of the global production.
Since the phosphate price increased rapidly in 2007, several new deposits have been explored to boost production. One frontier region is Morocco, where in the national Moroccan Phosphates Company (OCP) stated in 2010 that they were to almost double production to 55 Mt/year by 2020, by opening four new mines (OCP 2010). However, the annual report from 2011 is not as ambitious and expects an increase of 20 Mt by 2020 (OCP 2011). Other recent mining developments are taking place worldwide. In Namibia and New Zealand, companies want to start marine mining of phosphate rock sediment. New mines are also planned to be developed in Finland (1.5 Mt/year), Kazakhstan (1.0 Mt/year) and Saudi Arabia (1.5 Mt/year) (De Ridder et al., 2012).
In 2011, only about 17% of the produced phosphate rock concentrate was exported directly, with the largest share being upgraded to phosphoric acid or phosphate fertilizers (IFA 2014). The total global trade of all forms of phosphate was only 22.5 Mt P2O5 in 2011 (OCP 2011), which means that around 63% of the phosphorus was consumed locally in the producing countries. Of the global phosphorus trade, 9.8 Mt P2O5(43%) was in the form of phosphate rock (IFA 2014). Especially China and the United States consume most of their production domestically, why Morocco accounts for the bulk of the world’s exports to the countries dependent on imports and provides 36.7% of the global export market (OCP 2011). Following their expansion plans, Moroccan export share are expected to increase substantially.
This study makes no attempts to project future demand of phosphate rock. Some of the results in the models presented reach phosphate rock production many times higher than current levels, and depending on the development of factors such as global population, diets, efficiency and recycling of phosphorous, the demand of virgin phosphate rock can look very different in the future.
There are many potential bottlenecks that could limit production flows and these should be addressed in the context of the individual countries where the production is supposed to happen. If countries such as Morocco are expected to increase their phosphate rock production with several times the current production, issues such as local environmental impacts, water availability, access to energy and geopolitical issues should be addressed on a regional level. Also, food producers could become more or less completely dependent on phosphate rock from Morocco due to its large and increasing share of the export market, which could lead to something resembling a monopolistic situation for Morocco in the future with associated risks (Elser and Bennett, 2011).
One important aspect is that the countries responsible for the phosphate rock mining will inevitably face local environmental impacts. Most phosphate rock is mined using large scale surface mining, which tend to have large impacts on the environment as it disturbs local landscape, and a wide range of other local environmental impacts can follow, such as water contamination, air emissions, noise and waste generation (UNEP, 2001).
Both phosphate rock production and beneficiation is highly water intensive, and water scarcity may essentially limit the beneficiation in dry areas (Van Kauwenbergh 2010). Many countries that produce phosphate rock, such as countries in the MENA region, already suffer from a shortage of fresh water (De Ridder 2012). In Morocco, most water is currently used for agriculture, out of which 30% is taken from the groundwater, often in an unsustainable way, and the groundwater table has fallen by an average of 1.5 meters per year since 1969 (UNEP, 2009). Energy intensive desalination plants are built by the national Moroccan phosphates company OCP to meet their need for water (OCP 2011).
Access to energy, especially oil, has been pointed out as a potential problem in the future as mining and fertilizer production rely on cheap oil and higher oil prices with associated supply chain effects caused by peak oil could increase production costs (Cordell 2009; Fantazzini 2011; Hanjra and Qureshi, 2010). For individual countries to be able to produce large amounts of phosphate rock, or products based on phosphorus, they will need large amounts of oil.
Geopolitical problems and civil unrest is a potential issue for phosphate rock production. The Arab spring, starting at the end of 2010, affected phosphate rock supply as production fell in several important producing countries in the MENA region, and the protracted conflict in Syria continues to influence supply of phosphate (de Ridder et al., 2012). Although not affected much by the Arab spring, Morocco has seen strikes and protests over employment and wealth equity concerns, but has not experienced any major disruptions in production (Wellstead 2012). Another issue is that Western Sahara, currently contributing with about 10% of Morocco’s phosphate rock production, has been occupied by Morocco since 1975, creating a ground for potential future instabilities (Edixhoven 2013).
Phosphate rock of different origin varies widely in composition and contains different quantities of impurities, such as cadmium (IFA 1998). It is have been suggested that the high cadmium content in many phosphate rock deposits in Morocco may provide an incentive for declining demand for Moroccan phosphate rock in the future (De Ridder 2012).
The future of phosphate rock
The current waste of phosphorus fertilizer causes a great deal of environmental problems, and it is questionable if it is a good idea to extract all the phosphate rock reserves if it would still end up in lakes, streams and the sea. As more and more phosphorus have been added to the ecosystem, many lakes and coasts have seen an increased algae growth (De Ridder 2012), which in some cases have led to serious eutrophication and dead zones due to lack of oxygen (Ashley 2011; Elser 2012). Carpenter (2011) even considers that the planetary boundaries for eutrophication of freshwater due to phosphorus have already been exceeded. It is possible to recycle phosphorus from human excreta, manure and different types of waste products (Cordell 2011b) and efficiency improvements in production and usage of phosphorus could offer a possibility to postpone a potential production peak (Cordell 2013).
Perhaps most importantly, the rather enormous phosphate rock reserves in Morocco is not a reason enough to stop attempting to find solutions towards a more sustainable food and energy system.
Estimates of future production of phosphate rock vary greatly depending on models used and assumptions made. Simple curve fitting modelling on aggregated global production data constrained by current USGS reserve estimates suggest that global phosphate rock production could reach a peak about 70 years from now, while other models or other reserve estimates used paints widely different pictures.
References
Ashley, K., Cordell, D., Mavinic, D., 2011. A brief history of phosphorus: From the philosopher’s stone to nutrient recovery and reuse. Chemosphere 84, 737–746.
Bardi, U., 2005. The mineral economy: a model for the shape of oil production curves. Energy Policy 33, 53–61.
Bardi, U., 2009. Peak oil: The four stages of a new idea. Energy 34, 323–326.
Bardi, U., Lavacchi, A., 2009. A Simple Interpretation of Hubbert’s Model of Resource Exploitation. Energies 2, 646–661.
BGS, 2013. World mineral statistics archive. British Geological Survey. Available at: http://www.bgs.ac.uk/mineralsuk/statistics/worldArchive.html
Buckingham, D.A., Jasinski, S.M., 2012. Historical Statistics for Mineral and Material Commodities in the United States; Phosphate rock statistics. http://minerals.usgs.gov/minerals/pubs/historical-statistics/ds140-phosp.pdf
Carpenter, S.R., Bennett, E.M., 2011. Reconsideration of the planetary boundary for phosphorus. Environ. Res. Lett. 6, 014009.
Cooper, J., Lombardi, R., Boardman, D., Carliell-Marquet, C., 2011. The future distribution and production of global phosphate rock reserves. Resources, Conservation and Recycling 57, 78–86.
Cordell, D., Drangert, J.-O., White, S., 2009. The story of phosphorus: Global food security and food for thought. Global Environmental Change 19, 292–305.
Cordell, D., White, S., Lindström, T., 2011a. Peak phosphorus: the crunch time for humanity? The Sustainability Review. Available from http://thesustainabilityreview.org/peak-phosphorus-thecrunch-time-for-humanity/
Cordell, D., Rosemarin, A., Schröder, J.J., Smit, A.L., 2011b. Towards global phosphorus security: A systems framework for phosphorus recovery and reuse options. Chemosphere 84, 747–758.
Cordell, D., White, S., 2011c. Peak Phosphorus: Clarifying the Key Issues of a Vigorous Debate about Long-Term Phosphorus Security. Sustainability 3, 2027–2049.
Cordell, D., White, S., 2013. Sustainable Phosphorus Measures: Strategies and Technologies for Achieving Phosphorus Security. Agronomy 3, 86–116.
De Ridder, M., De Jong, S., Polchar, J., Lingemann, S., 2012. Risks and Opportunities in the Global Phosphate Rock Market: Robust Strategies in Times of Uncertainty. The Hague Centre for Strategic Studies (HCSS). Report No 17 | 12 | 12. ISBN/EAN: 978-94-91040-69-6
Déry, P., Anderson, B., 2007. Peak phosphorus. Resilience. Available from:http://www.resilience.org/stories/2007-08-13/peak-phosphorus
EcoSanRes, 2008. Closing the Loop on Phosphorus. EcoSanRes Factsheet 4. Stockholm Environment Institute. Available at: http://www.ecosanres.org/pdf_files/ESR-factsheet-04.pdf
Edixhoven, J.D., Gupta, J., Savenije, H.H.G., 2013. Recent revisions of phosphate rock reserves and resources: reassuring or misleading? An in-depth literature review of global estimates of phosphate rock reserves and resources. Earth Syst. Dynam. Discuss. 4, 1005–1034.
Elser, J., Bennett, E., 2011. Phosphorus cycle: A broken biogeochemical cycle. Nature 478, 29–31.
Elser, J.J., 2012. Phosphorus: a limiting nutrient for humanity? Curr. Opin. Biotechnol. 23, 833–838.
Evenson, R.E., Gollin, D., 2003. Assessing the Impact of the Green Revolution, 1960 to 2000. Science 300, 758–762.
Fantazzini, D., Höök, M., Angelantoni, A., 2011. Global oil risks in the early 21st century. Energy Policy 39, 7865–7873.
Filippelli, G.M., 2011. Phosphate rock formation and marine phosphorus geochemistry: The deep time perspective. Chemosphere 84, 759–766.
Fixen, P.E., 2009. World Fertilizer Nutrient Reserves— A View to the Future. Better Crops, Vol. 93 No. 3 pp. 8-11.
Gilbert, N., 2009. Environment: The disappearing nutrient. Nature News 461, 716–718. Published in Resources Conservation and Recycling Volume 93, December 2014, Pages 178–187 http://dx.doi.org/10.1016/j.resconrec.2014.10.011
GPRI, 2010. GPRI Statement on Global Phosphorus Scarcity. http://phosphorusfutures.net/files/GPRI_Statement_responseIFDC_final.pdf
Hanjra, M.A., Qureshi, M.E., 2010. Global water crisis and future food security in an era of climate change. Food Policy 35, 365–377.
Herring, J.R., Fantel, R.J., 1993. Phosphate rock demand into the next century: Impact on world food supply. Nonrenewable Resour. 2, 226–246.
Hubbert, M.K., 1956. Nuclear energy and the fossil fuels. Report No. 95, Shell Development Company.
Hubbert, M.K., 1959. Techniques of prediction with application to the petroleum industry., Published in 44th Annual Meeting of the American Association of Petroleum Geologists. Shell Development Company, Dallas, TX.
Höök, M., Bardi, U., Feng, L., Pang, X., 2010. Development of oil formation theories and their importance for peak oil. Marine and Petroleum Geology 27, 1995–2004.
Höök, M., Li, J., Oba, N., Snowden, S., 2011. Descriptive and Predictive Growth Curves in Energy System Analysis. Natural Resources Research 20, 103–116.
IFA, 1998. The Fertilizer Industry, Food Supplies and the Environment. International Fertilizer Industry Association and United Nations Environment Programme, Paris, France.
IFA, 2011. Global Phosphate Rock Production Trends from 1961 to 2010. Reasons for the Temporary Set-Back in 1988-1994. International Fertilizer Industry Association (IFA). Paris, France.
IFA, 2014. Production and trade statistics. International Fertilizer Industry Association. http://www.fertilizer.org/ifa/HomePage/STATISTICS/Production-and-trade
Jakobsson, K., Bentley, R., Söderbergh, B., Aleklett, K., 2012. The end of cheap oil: Bottom-up economic and geologic modeling of aggregate oil production curves. Energy Policy 41, 860–870.
Jasinski, S.M., 2013a. Annual publication: Mineral Commodity Summaries: Phosphate Rock (1996-2013) U.S. Geological Survey. http://minerals.usgs.gov/minerals/pubs/commodity/phosphate_rock
Jasinski, S.M., 2013b. Minerals Yearbook, Phosphate Rock (1994-2013). U.S. Geological Survey. http://minerals.usgs.gov/minerals/pubs/commodity/phosphate_rock/
Koppelaar, R.H.E.M., Weikard, H.P., 2013. Assessing phosphate rock depletion and phosphorusrecycling options. Glob. Environ. Change, 23(6): 1454–1466.
Krauss, U.H., Saam, H.G., Schmidt, H.W., 1984. International Strategic Minerals Inventory SummaryReport – Phosphate, U.S. Geological Survey Circular 930-C. U.S. Department of the Interior.
Liu, X., et al. 2016. Intensification of phosphorus cycling in China since the 1600s. Proceedings of the National Academy of Sciences.
May, D., Prior, T., Cordell, D., Giurco, D., 2012. Peak minerals: theoretical foundations and practicalapplication. Natural Resources Research, 21(1):43-60.
Mohr, S., Evans, G., 2013. Projections of Future Phosphorus Production. Philica.com. URLhttp://philica.com/display_article.php?article_id=380
Mohr, S., Höök, M., Mudd, G., Evans, G., 2011. Projection of long-term paths for Australian coal production—Comparisons of four models. International Journal of Coal Geology 86, 329–341.
Mórrígan, T., 2010. Peak Phosphorus: A Potential Food Security Crisis. Global & International Studies, University of California. Santa Barbara, CA.
OCP, 2010. OCP Annual Report 2010. Office Chérifien des Phosphates.
OCP, 2011. OCP Annual Report 2011. Office Chérifien des Phosphates.
Pan, G., Harris, D.P., Heiner, T., 1992. Fundamental issues in quantitative estimation of mineral resources. Nat Resour Res 1, 281–292.
Rosemarin, A., 2004. The precarious geopolitics of phosphorous. Down to Earth. http://www.downtoearth.org.in/content/precarious-geopolitics-phosphorous
Rosemarin, A., De Bruijne, G., Caldwell, I., 2009. Peak phosphorus: the Next Inconvenient Truth. The Broker. http://www.thebrokeronline.eu/Articles/Peak-phosphorus
Scholz, R.W., Ulrich, A.E., Eilittä, M., Roy, A., 2013. Sustainable use of phosphorus: A finite resource. Science of The Total Environment 461–462, 799–803. Published in Resources Conservation and Recycling Volume 93, December 2014, Pages 178–187 http://dx.doi.org/10.1016/j.resconrec.2014.10.011
Scholz, R.W., Wellmer, F.-W., 2013. Approaching a dynamic view on the availability of mineral resources: What we may learn from the case of phosphorus? Global Environmental Change 23, 11–27.
Schröder, J.J., Cordell, D., Smit, A.L., Rosemarin, A., 2009. Sustainable Use of Phosphorus. Plant Research International, Wageningen UR and Stockholm Environment Institute (SEI), Report 357.
Smil, V., 2000. Phosphorus in the Environment: Natural Flows and Human Interferences. Annual Review of Energy and the Environment 25, 53–88.
Smit, A.L., Bindraban, P.S., Schröder, J.J., Conijn, J.G., Van der Meer, H.G., 2009. Phosphorus in the agriculture: global resources, trends and developments. Plant Research International, Wageningen UR. Report No. 282.
Steen, I., 1998. Phosphorus availability in the 21st century: Management of a non-renewable resource. Phosphorus & Potassium, Issue No:217. Available from: http://www.nhm.ac.uk/researchcuration/research/projects/phosphate-recovery/p&k217/steen.htm
Sverdrup, H.U., Ragnarsdottir, K.V., 2011. Challenging the planetary boundaries II: Assessing the sustainable global population and phosphate supply, using a systems dynamics assessment model. Appl. Geochem. 26, Supplement, S307–S310.
Udo de Haes, H.A., van der Weijden, W.J., Smit, A.L., 2009. Phosphate – from surplus to shortage. Steering Committee for Technology Assessment. Ministry of Agriculture, Nature and Food Quality, Utrecht.
UNEP, 2001. Environmental Aspects of Phosphate and Potash Mining. United Nations Environment Programme and International Fertilizer Industry Association, Paris, France.
UNEP, 2009. Agribusiness, Issue 1. Chief Liquidity Series. United Nations Environment Programme Finance Initiative.
UNEP, 2011. Phosphorus and Food Production, UNEP Year Book 2011.
UNEP/UNIDO, 2000. Mineral Fertilizer Production and the Environment. Part 1. The Fertilizer Industry’s Manufacturing Processes and Environmental Issues. United Nations Environment Programme Industry and the Environment United Nations Industrial Development Organization in collaboration with the International Fertilizer Industry, Paris, France.
USBM, 1993. Bureau of Mines Minerals Yearbook (1932-1993). http://minerals.usgs.gov/minerals/pubs/usbmmyb.html
USGS, 2013. Mineral Commodity Summaries 2013. Appendix C: Reserves and Resources. http://minerals.usgs.gov/minerals/pubs/mcs/2013/mcsapp2013.pdf
Vaccari, D.A., 2009. Phosphorus: A Looming Crisis. Sci. Am. 300, 54–59.
Vaccari, D.A., Strigul, N., 2011. Extrapolating phosphorus production to estimate resource reserves. Chemosphere 84, 792–797.
Van Enk, R.J., Van der Vee, G., Acera, L.K., Schuiling, R., Ehlert, P., 2011. The phosphate balance: current developments and future outlook. The ministry of Economic Affairs, Agriculture and nnovation initiated and finances InnovationNetwork. ISBN:978–90–5059–414–1
van Kauwenbergh, S., 2010. World Phosphate Rock Reserves and Resources.The International Fertilizer Development Center (IFDC).
van Vuuren, D.P., Bouwman, A.F., Beusen, A.H.W., 2010. Phosphorus demand for the 1970–2100 period: A scenario analysis of resource depletion. Glob. Environ. Change 20, 428–439.
Wellstead, J., 2012. Political Risks in MENA Phosphate Markets. Potash Invest. News. Available from: http://potashinvestingnews.com/4799-political-risks-mena-phosphate-markets-tunisiamorocco-jordan-syria-afric.html
Vikström, H., Davidsson, S., Höök, M., 2013. Lithium availability and future production outlooks.Applied Energy 110, 252–266.