Preface. Below is a review of “Prime Mover: A Natural History of Muscle: from Natural History Magazine.
Since both my books explain why we will be returning to biomass and muscle power, here’s yet another preparation opportunity: breed horses and animal and human powered treadwheels. Or generate electricity from the 54 million gym members…
Before fossil fuels, the energy to do work came from muscle power and the heat from burning biomass, mainly wood. When I visited the Deutsches museum in Munich in 2017, I saw two animal treadmills. The first picture shows a dog treadwheel in a nailmaker’s workshop in 1850. The dog ran inside the “drum” with the treadwheel operating the bellow to fan the smith’s fire. When more fire was needed, the nailer shouted at the dog to make it run more quickly to fan the fire more strongly. Dog treadwheels were used at nailmaker’s until about 1930.
The picture below is a treadmill used by either horses or oxen in 1900 to drive farm machinery. The treadmill comprises an endless belt of wooden planks. Oxen or horses are roped to it in such a way that they are strangled if they do not continually walk on the belt. The rotary movement is taken up by a pulley. With a small working load a centrifugal brake ensures that the belt does not run too quickly. Treadmills were also built for two animals working side by side and for small animals, such as dogs or goats.
As you can see below, water, wind, firewood, and animal and human muscle power provided little power compared to coal from 1561 to 1859.
Figure 1. Annual energy consumption per person (megajoules) in
England and Wales 1561-70 to 1850-9 and in Italy 1861-70.
Figure by Tony Wrigley, Cambridge University. Chart from Gail Tverberg's
post Rethinking Renewable Mandates
As fossil energy declines, muscle power will increasingly have to replace it. Or as Vaclav Smil wrote in “Energy and Civilization”: “The simplest way of transporting loads is to carry them. Where roads were absent people could often do better than animals: their weaker performance was often more than compensated for by flexibility in loading, unloading, moving on narrow paths, and scrambling uphill. Similarly, donkeys and mules with panniers were often preferred to horses: steadier on narrow paths, with harder hooves and lower water needs they were more resilient. The most efficient method of carrying is to place the load’s center of gravity above the carrier’s own center of gravity-but balancing a load is not always practical. In relative terms, people were better carriers than animals. Typical loads were only about 30% of an animal’s weight (that is, mostly just 50-120 kg) on the level and 25% in the hills. Men aided by a wheel could move loads far surpassing their body weight. Recorded peaks are more than 150 kg in Chinese barrows where the load was centered right above the wheel’s axle.”
Alice Friedemann www.energyskeptic.com Author of Life After Fossil Fuels: A Reality Check on Alternative Energy; When Trucks Stop Running: Energy and the Future of Transportation”, Barriers to Making Algal Biofuels, & “Crunch! Whole Grain Artisan Chips and Crackers”. Women in ecology Podcasts: WGBH, Planet: Critical, Crazy Town, Collapse Chronicles, Derrick Jensen, Practical Prepping, Kunstler 253 &278, Peak Prosperity, Index of best energyskeptic posts
***
Steven Vogel. 2003. Prime Mover: A Natural History of Muscle. W. W. Norton Reviewed in June 2004. Natural History Magazine
Today we have machines for making people move in place: run, walk uphill, push pedals back and forth or up and down, row, ski, or even climb a never-ending staircase. Machines that are designed to waste energy and that usually rely on still more energy, in the form of electricity, to run-who would have anticipated their popularity? Thanks to the ingenuity of these contraptions’ designers and purveyors (people who, one might say, live off the fat of the land), the toils of Sisyphus have been transformed into a healthful pastime.
Such machines mirror the ancient technology of animal-powered engines. Millennia ago, humans discovered that wheels could do something other than turn tables for shaping pottery or underpin a vehicle and that a domesticated animal could do something other than carry a load or pull a wagon. We figured out that animals could do lots of useful tasks by turning wheels that were fixed in place. In an era when the non-muscular power sources included only a few types of sails and waterwheels, such a realization was no small matter. The diverse devices that followed had in common a fixed position, rotational motion, and whole-animal muscle power. Few looked much like today’s fancy exercise machines. Some were designed to be powered by humans, others by (usually) domesticated animals, and a few by either (depending on availability) showing, perhaps, how little the inventors distinguished a serf, a slave, or a convict from other sources of involuntary labor.
We can recognize three major designs. In the oldest, a horizontal bar jutted from a vertical shaft. An animal attached to the bar turned the shaft by circling. Because the animal itself rotated, the machine needed no true crank, such as the kind we use to pedal bicycles. Sometimes the vertical shaft was itself part of the business end of the machine, as in systems that ground grain between stones. Sometimes a pair of gears connected the vertical shaft to a horizontal one, often to run a chain of buckets descending into a well.
In a second type, a human or a domestic animal worked within a huge hollow wheel, like a hamster in an exercise wheel. The motor thus climbed rather than pulled. A greater load on the wheel meant a greater resistance to its being turned, and this meant that the living motor had to climb farther up the inside of the wheel to keep it going. The increasing slope gave the wheel a neatly self-regulating character: the motor’s output was automatically matched with the load. Bipeds such as humans made particularly good motors, but these cage wheels didn’t require domesticated creatures-even bears, contend historians, could be pressed into service.
The third design also made an animal climb, but it used a moving sloped platform instead. This ancestor of our fitness treadmills presented severe mechanical challenges: its sliding platform had to support both an animal’s weight and the impact of its feet, as well as be durable and sufficiently flexible to go around revolving drums fore and aft. But the challenges were worth overcoming: its slope could be adjusted to match the motor with the load, and the variable slope made the machine less finicky about what sort of animal powered it. However, the engineering feats required to build such true treadmills meant that they were uncommon before the 19th century.
Not that the three basic designs exhausted the possibilities for muscle-powered engines: A person could sit on a plank and rotate an inclined disk by pushing the edge with his feet, or an animal could walk in place uphill on top of the disk. A person could pull downward on crossbars on the outside of a revolving vertical wheel (essentially replacing water on a waterwheel) or pull on a slack chain hung over such a wheel. Indeed, the makers of modern exercise equipment might profitably peruse the works of great Renaissance engineers such as Georgius Agricola, Agostino Ramelli, and Fausto Veranzio when deciding what new kinds of devices to unleash on the public.
Although muscle-powered engines are ancient, they originated long after chariots, carts, and potters’ wheels-probably about 4,000 years ago, in the Middle East or India. Their main use in that part of the world was (and remains) lifting water with a bucket chain, a task that demanded a reliable way to turn horizontal into vertical motion. The problem’s solution-putting a pair of gears at right angles to each other-was far from obvious, and this may have delayed its invention or adoption. Rotary grain mills needed no such gears, but these mills came into use later, perhaps initially invented by the Greeks about 400 B.C. By the first century A.D, the Romans used slaves and donkeys to power a highly effective version-their mola asinaria (asinine, or donkey, mill).
Revolving cage wheels may have been a Roman innovation. Unambiguous images of them occur on bas-reliefs, and remnants of the actual wheels have been found at Pompeii. As with rotary grain mills, humans and donkeys provided the energy. Such wheels powered cranes that lifted blocks into place during the building of tall structures. Centuries later, similar cranes helped a more technologically savvy culture erect the great medieval cathedrals.
Because wells and irrigation systems were widespread and the hulls of wooden ships usually leaked, pumping water was a common use of stationary muscle-driven engines. Sometimes these engines were used to lift water to heroic heights. In one Roman mine, a cascade of eight pairs of scoop wheels raised water almost a hundred feet. Grinding grain and lifting stonework were not these engines’ only other tasks; in medieval Europe they also ran sawmills, pile drivers, dough-kneading machines, dockside cranes, bellows, and even one wheel (powered by a dog) that turned a roasting spit.
Muscle-powered mechanical engines also provided an alternative to sails and oars for the propelling of boats. On China’s rivers, human-powered cage wheels drove paddle wheelers as long ago as the eighth century A.D, though this mating of two relatively efficient devices never caught on in the West. Here shipbuilders stuck with oars, but building large oar-powered ships proved difficult: no matter how many were added to power a bigger ship, the oars couldn’t keep pace with the increased drag of the vessel. Also, larger oars were heavy and clumsy to maneuver and required multiple oars-men. By contrast, paddle wheelers lose nothing by being big, and it’s easy to link the paddle wheel to the cage wheel amidships.
One form of animal-powered boat did appear in the West, mainly in eastern North America, during the first half of the nineteenth century. Shortly after steamboats came into use, horse-powered “teamboats” began serving as ferries across waterways such as New York’s Hudson River. They were less expensive than steam, more reliable than sail, and required none of the human labor–more scarce in the New World than in the Old–demanded by oars. In one design, two or more horses walked in a circle on deck, turning a capstan amidships that was geared to a paddle wheel set between a pair of catamaran-like hulls. Another version had a turntable below deck, with horses in fixed stalls and two paddle wheels, one on each side of a single hull.
Usually, however, the nineteenth century’s many muscle-powered engines operated away from water: in prisons and on the prairie. Penal versions abounded. In its entry for “treadmill,” the great Oxford English Dictionary, compiled at the century’s end, recognized only a penal application for the machines. Punishment and useful work-what a nice combination. British parliamentary commissions repeatedly examined and endorsed the treadmill’s safety and beneficial effects on the health of inmates.
Great Britain was not alone in using these mills as a correctional device. New York City began using one in Bellevue Penitentiary in about 1820. Eight prisoners climbed the wide rows of steps that formed a revolving drum, while four sat in reserve. Each team member (some were women) therefore worked two-thirds of the time. Still, treadmills remained relatively uncommon in America; the shortage of labor meant that humans were rarely employed as motors when other animals would do.
Used judiciously, however, a muscle-powered engine provided aerobic exercise to incarcerated people who otherwise would have lacked it. Furthermore, the engines supplied necessities, such as ground grain for prison bakeries. An 1824 report (“The History of the Treadmill,” by James Hardie) makes much of the safety of the New York wheel, and its assertions about the health of inmates using it don’t seem unreasonable. Inmates apparently received sufficient food for the work. But its punitive character is evident: the jailers claimed rapid attitude adjustments in formerly obstreperous prisoners. As Thomas Henry Huxley, famous defender of Charles Darwin, put it when complaining about an adversary, “I would willingly agree to any law which would send him to the treadmill.”
For size and technological audacity, nothing has ever come close to the agricultural machines used during the nineteenth century on the North American prairie. After about 1880, large “combines,” pulled by up to forty horses, reaped and threshed wheat as they moved through the fields. But in the preceding decades, the threshing of grain depended on stationary machines called sweeps, powered by as many as twenty horses pulling radial bars as they circled. The machine’s parts were brought to a threshing location the evening before the scheduled work was begun, and the thresher was assembled before dawn; after being run all day, it was dismantled and moved (by its own horses) to the next farm. While these big and otherwise sophisticated machines incorporated a novel level of portability, they also relied on the oldest of designs for muscle-powered engines.
Unlike the combines, most nineteenth-century American devices were relatively small, general- purpose machines powered by one to four horses or oxen. These often used the third basic design, a suspended treadmill of two rollers and an inclined “belt.” The belt consisted of wooden boards, laid perpendicularly to the animals’ path and hinged side-to-side, forming an endless moving platform that slid on greased tracks or small rollers positioned between the big front and rear rollers. A one- or two-animal model looked like an open horse trailer. This machine worked much like a modern tractor motor; it could be connected to various machines and could be pulled out of the barn and positioned wherever it was needed for chores such as sawing logs. These multipurpose units appeared in farm catalogs as late as 1890.
Smaller versions of the giant threshing sweeps also appeared as late as 1890. For some tasks, either a treadmill or a sweep was suitable. Sweeps, which were simpler and lighter relative to their potential power, demanded more goading of the driving animals, and many designs required that the animals step over a horizontal drive-shaft once during every revolution. Treadmills, which were more compact and easier to move and put to work than the sweeps, were harder on the animals’ hooves. But treadmills were advertised as being twice as effective, which was not an unreasonable claim: on an inclined belt, an animal works by lifting itself; attached to a sweep, a harnessed animal pulls-a less natural activity, severely constrained by the effectiveness of the harness.
Like other muscle-powered machinery, treadmill devices were not designed only for horses or oxen. Household (or dooryard) models even included a butter churn; the manufacturer asserted that dogs, goats, sheep, or children could power it.
Such devices demonstrate that mechanization needn’t involve muscle-displacing motorization. Nonetheless, by the end of the century, steam power had largely replaced muscle power. The small steam engines of the nineteenth century may have been less fuel-and weight-efficient than gasoline-powered engines, but in low-population areas they were a good solution. Engines in which steam pushed pistons operated at relatively low pressures and temperatures, so they could be built simply, of common metals, and were easy to repair. Furthermore, they could burn anything combustible. Farm museums across North America still display such steam engines-self-propelled tractors that could also work as stationary power sources, powering all the same accessories as the treadmills that preceded them.
How good were these ingenious animal-powered machines at making muscle do useful work?
Where humans worked against gravity, as they did inside cage wheels and upon treadmills, we can calculate the power outputs. As a benchmark, we might use data, first obtained in the eighteenth century by British scientists Jean Desaguliers and John Smeaton, of the power a human laborer could produce if working steadily all day: 90 to 100 watts. In contemporary terms, this means that if you attach a generator to an exercise machine, you can watch TV as long as you climb, pedal, or row.
By the same token, a Roman cage wheel sixteen feet in diameter and eight feet across accommodated six to eight men, who could, forty times per hour, jointly lift one ton a distance of twenty-seven feet-which equals a power output of 600 foot-pounds per second. Dividing that among eight workers, we calculate a power output per person of just over 100 watts. That figure attests both to respectable efficiency for the machine and to considerable effort for the workers (who may have worked in relays).
On the Bellevue Penitentiary treadmill, prisoners climbed on treads protruding from a wheel that was slightly over five feet in diameter and turned three times each minute. If one assumes that a typical prisoner weighed 132 pounds, then the prisoner must have worked at a power of almost 140 watts. Since the normal duty cycle allowed each prisoner to rest one-third of the time, the sustained output would have been a little over 90 watts-sustained, according to the report, for up to ten hours a day. That figure of 90 watts confirms the reported unpleasantness of the task. A similar output was demanded of nineteenth-century Australian convicts, who worked up to twelve hours per day; some said they’d rather hang than work their mill.
We can view that 90 watts in yet another context. At best, only about one-fourth of the energy in food emerges as useful mechanical work. Thus, laboring on the treadmill-sustaining 90 watts for ten hours-itself requires more than 3,000 Calories. So Bellevue’s inmates worked hard enough and long enough to require double the food intake of a normally active adult male.
Although nonhuman animals don’t complain and can be fed cheaper food, attaching them to machines greatly lessens their efficiency. Which animals give the best service? Biology tells us that bigger is better. The strength and power of muscle tissue vary little from animal to animal, and mammals all have about the same amount of muscle: about 40 percent of body weight. But larger creatures spend relatively less energy on basic body functions, and this increases the fraction of their food that can be appropriated for labor-so forget battalions of wheel-turning rodents. Insects put out lots of power for long periods while spending less on personal maintenance than even big mammals do; in particular, they don’t insist on staying warm when idle. Under laboratory conditions, flying insects such as fruit flies and migratory locusts have powered stationary engines with their beating wings. But even large insects remain impractically small for our purposes.
Nor can practicality be ignored at the other end of the scale. Elephants, whatever their potential efficiency, are awkwardly large. Oxen have given excellent service for millennia, and horses for a little more than a thousand years-since the invention of the horse collar, which enabled them to pull effectively-but only on sweeps and treadmills. Few if any tractable animals come close to the mechanical versatility of agile humans.
Today, only a few types of muscle-powered stationary engines remain in use. A “treadle pump” first disseminated in Bangladesh during the 1980s and now used by many farmers in Asia and sub-Saharan Africa) pumps water for irrigation; it’s run by a person who climbs what looks like a Stair-Master stair climber. More sophisticated and convenient I means of generating energy have largely taken the place of muscle-powered machinery Engines powered by fossil fuels require far less infrastructure than do working animals and come in a much wider i range of sizes and models. Still, why not take instruction from history and hook a generator to your exercise bike or rowing machine? That power source could run some entertainment device that performs only when you do likewise.
You Expect Me to Do Watt?
A worker doing hard physical labor all day long-or a felon turning a treadmill-can put out about 100 watts of power. That’s the output, in the form of a little light and a lot of heat, of the familiar light bulb. It’s a little more than the rate at which an inactive human heats a room. But to understand 100 watts of muscle power, one needs to turn to a quantifiable everyday task that humans do with reasonable efficiency. Climbing stairs fits that bill.
When you climb a flight of stairs, what’s your power output? Just multiply your weight in pounds by the height of each step in inches and by your climbing rate in steps per second, and then divide by 9. The last number takes care of gravity and converts the figure into watts. I weigh 140 pounds. When climbing seven-inch steps at two per second, I put out about 220 watts-a rate that I, an age-challenged man, can sustain only briefly. Ascending a down escalator, I work at 140 watts.
But what climbing rate corresponds to an output of 100 watts? Divide 900 by your weight in pounds and by the step height in inches; the resulting figure is how many steps per second you would have to climb. On seven-inch stairs, I’d have to ascend them at a little less than one step per second-trivial for the first couple of flights but a tiring regimen to keep up for even an hour.
What about fuel? We’re at best only about 25 percent efficient, so an output of 100 watts requires a minimum input of 400 watts, which translates (when we multiply by 0.86) into about 350 Calories per hour. Burning a tenth
1 pound of good fuel-fat-yields 350 Calories, so working at 100 watts for eight hours costs less than a pound of body fat-still nearly double a human male’s normal energy use.
By any technological yardstick, we animals, whether horses or humans, are strange engines. For a difficult task of only a few seconds’ duration, a person can put out thousands of watts–many times the 746 watts in one official horsepower. For tasks lasting a few minutes, a fit human can generate perhaps 1,000 watts. For an activity that must be sustained for an hour, output drops to around 300; for an activity kept up all day, 150 watts is about the maximum. But by physiological standards, horses and humans (and dogs but not cats) sustain especially high power outputs. Fleet-footed ancestors bequeathed us the lungs and hearts that let us work long and hard.
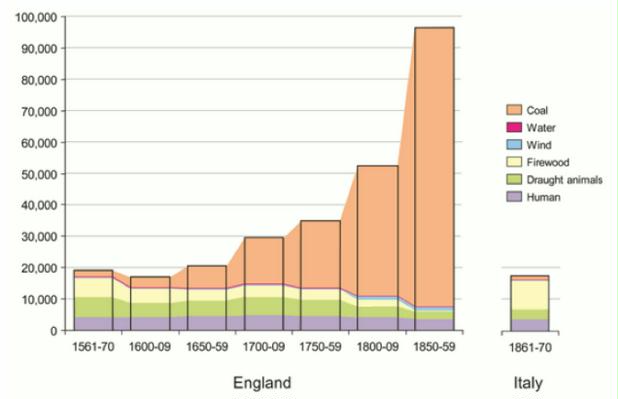
3 Responses to Muscle Power