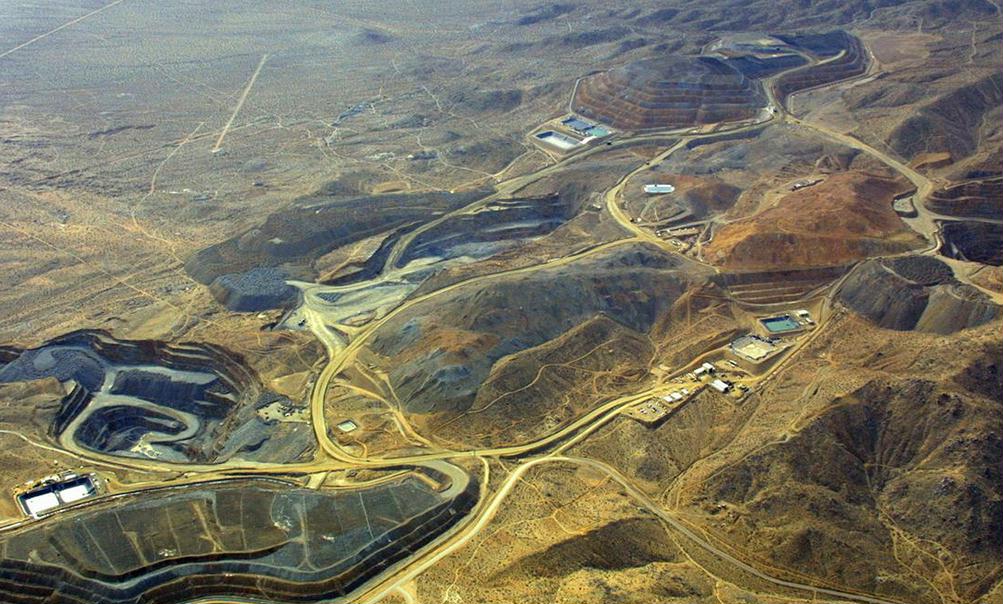
Preface. Electricity generating contraptions like wind and solar can’t replace the 50% of oil used in global manufacturing, because they can’t generate the high heat needed, or run battery or catenary electric trucks as I explained in “When Trucks Top Running”. Nor are there enough rare earth metals, lithium, cobalt and others to scale up renewables.
Peak metals in the news:
Tabuchi H (2021) Thieves Nationwide Are Slithering Under Cars, Swiping Catalytic Converters. The pollution-control gadgets are full of precious metals like palladium, and prices are soaring as regulators try to tame emissions. Crooks with hacksaws have noticed. New York Times.
And there simply aren’t enough minerals on earth to make a transition to “renewables”:
- 2021 All the Metals We Mined in One Chart Visualcapitalist.com
- Antonio Turiel (2021) Some inconvenient questions: An open letter
- Simon P. Michaux (2021) The mining of Minerals and the Limits to growth video
Alice Friedemann www.energyskeptic.com Women in ecology author of 2021 Life After Fossil Fuels: A Reality Check on Alternative Energy best price here; 2015 When Trucks Stop Running: Energy and the Future of Transportation”, Barriers to Making Algal Biofuels, & “Crunch! Whole Grain Artisan Chips and Crackers”. Podcasts: Crazy Town, Collapse Chronicles, Derrick Jensen, Practical Prepping, KunstlerCast 253, KunstlerCast278, Peak Prosperity
***
Since renewables depend on fossil fuels entirely for every step of their life cycle, I call them Rebuildables. The showstoppers keeping them from replacing fossil fuels are that they can’t substitute for fossils in either transportation (explained in When Trucks Stop Running), or in manufacturing (explained in “Life After Fossil Fuels”, because they can’t generate the high heat needed to smelt and forge metals, ceramics, solar cells, and the other components they’re made of. Without transportation and manufacturing, they can’t make “babies” and reproduce, no matter how much electricity they generate. And given their fossil-dependent life cycle, their energy return is certainly negative.
In addition, there aren’t enough rare earth metals, platinum group metals, steel, copper, and so on to scale them up to replace fossil fuels.
Just look at the materials to make a 2 MW wind turbine. To generate just half of U.S. electricity with wind would require 1,095,000 2 MW wind turbines (Friedemann 2015), each of them requiring 1,671 tons of material, including 1300 tons concrete, 295 tons steel, 48 tons iron, 24 tons fiberglass, 4 tons copper, and Chinese rare earth metals 0.4 tons of neodymium and .065 tons dysprosium (Guezuraga 2012, USGS 2011). Then rinse and repeat every 20 years with 3.7 trillion pounds of materials.
Utility scale batteries simply don’t scale up. The world’s largest lithium-ion battery project, a 300 MW facility, is being built in Moss Landing, California, adding enough storage capacity to the grid to supply about 2,700 homes for a month (or to store about .0009 percent of the electricity the state uses each year). But they’re far too expensive and don’t last long (15 year lifespan). At best, batteries can step in when peak power is needed, which mainly natural gas plants do today. They can not fill in the gaps of days, weeks, and even months when wind and solar generation flags. Especially in California, where both solar and wind fall off greatly in the fall and winter (Temple 2018).
Add billions more tons of materials to the rebuildable power shopping list for transmission, power plants, hundreds of square miles of backup utility-scale batteries, and then replace them in 20-25 years.
Using fossil energy every step and releasing a lot of CO2, since mining consumes 10% of world energy (TWC 2020).
If you can get these minerals that is. By mid-century many minerals and metals needed for high-tech could be running short , including stainless steel, copper, gallium, germanium, indium, antimony, tin, lead, gold, zinc, strontium, silver, nickel, tungsten, bismuth, boron, fluorite, manganese, selenium, and more (Pitron 2020 Appendix 14, Sverdrup 2019, Kerr 2012 and 2014, Frondel 2006, Barnhart 2013, Bardi 2014, Veronese 2015).
Computers are made of 60 minerals, many quite rare with no substitutable elements (EC 2017, NRC 2008, Graedel 2015). Fortunately the abacus can be made entirely with renewable wood.
Bardi (2014) wrote “The limits to mineral extraction are not limits of quantity; they are limits of energy. Extracting minerals takes energy, and the more dispersed the minerals are, the more energy is needed. Today, humankind doesn’t produce sufficient amounts of energy to mine sources other than conventional ores, and probably never will. But long before they “run out”, if oil peaks, then game over, fossil fuel resources are necessary for the extraction of almost everything else, and the easy high-grade ores have been mined, leaving crummy ore and expensive declining fossils left to extract it.”
We’re out of time. It would take 15 years to ramp up rare earth mining to prevent China from controlling most of these 17 metals (GAO 2010). Though all China has to do is drop the price of a metal to drive a mine out of business.
Not a problem many say. We’ll recycle. But some elements are impossible to pry out of composite materials and alloys (Bloodworth 2014, Hageluken 2012). The cost to recover most rare metals exceeds their value. Recycling is time-consuming and uses toxic chemicals to separate them out. Of the 60 most used industrial metals, 34 recycled less than 1% of the time, and another eight less than half the time (UNEP 2011).
Solar panels don’t last forever. Ninety percent of solar panels are going into the landfill or are sent to Europe. They are not being recycled in the U.S. because it costs more, isn’t required and is expensive to dissemble, etch, and melt them to remove lead, cadmium, copper, gallium, aluminum, glass, and silicon solar cells.
While some parts of a wind turbine can be recycled, 720,000 tons of blade material are expected to end up in landfills over the next 20 years, especially the up to 300-foot-long blades made of materials not worth salvaging: resin and fiberglass (Stella 2019).
And it is not just solar, wind, nuclear, and other alternatives that use rare metals. The entire universe of green energy depends on dozens of metals (i.e. rare earth, platinum) as alloys in steel, electronics, computers, the power grid, phones, wind turbines, magnets, photovoltaic cells, electric motors, satellites, semi-conductors, telecommunications, fuel cells, batteries, lasers, fiber optics, catalysts, aluminum alloys, integrated circuits, GPS navigation, and much more.
We are running out of time. China produces 90% of rare earth metals, and nearly all of dozens of other metals, plus owns part of all of mining companies around the world. It’s as if Saudi Arabia bought most of the world’s oil fields.
The Chinese now control the entire manufacturing chain from mining to metals to making missiles, components used in defense systems, communications, computers and more. This is driving calls in the U.S. and Europe to open their own rare metal mines lest a missile using Chinese parts be programmed to fail in war.
Why compete? Let China mine for essential minerals. Mining and ore processing are the second most polluting industry on earth, spewing out acid rain and heavy metals onto land, water, and air (PEBI 2016). One fifth of China’s arable land is polluted from mining and industry (Chin 2014). If their high-technology parts are booby-trapped to prevent war, all the better. Why waste rapidly declining oil on wars?
Green energy is anything but clean and green, and quite a Pyrrhic victory for China!
References
Andrews R. 2015. Renewable energy storage and power-to-methane. Energy Matters.
ASCE. 2017. America’s infrastructure report card. Energy D+. American society of civil engineers.
Bardi U. 2014. Extracted: How the Quest for Mineral Wealth Is Plundering the Planet. Chelsea Green.
Barnhart C, et al. 2013. On the importance of reducing the energetic and material demands of electrical energy storage. Energy Environment Science 2013(6): 1083–1092.
Bloodworth A. 2014. Track flows to manage technology-metal supply. Recycling cannot meet the demand for rare metals used in digital and green technologies. Nature 505: 19-20.
Cartlidge E. July 11, 2017. Fusion energy pushed back beyond 2050. BBC.
CE. 2020. Solar energy generation by state. ChooseEnergy.com.
CEC. 2014. Estimated cost of new renewable and fossil generation in California. California Energy Commission.
CCST. 2011. California’s energy future: The view to 2050 summary report. California Council on Science & Technology.
CCST. 2012. California’s energy future: electricity from renewable energy and fossil fuels with carbon capture and sequestration. California: California Council on Science and Technology.
Chin J, et al. 2014. China details vast extent of soil pollution. About a fifth of nation’s arable land is contaminated with heavy metals. Wall Street Journal.
Cooper M. 2013. Renaissance in reverse: competition pushes aging U.S. nuclear reactors to the brink of economic abandonment. Institute for Energy & the environment, Vermont Law School.
Davidsson S, et al. 2012. A review of life cycle assessments on wind energy systems. The International Journal of Life Cycle Assessment.
Davidsson S, et al. 2014. Growth curves and sustained commissioning modelling of renewable energy: Investigating resource constraints for wind energy. Energy Policy.
De Decker K. 2011. The bright future of solar thermal powered factories. Low Tech magazine.
Dittmar M. 2011. The end of cheap uranium. Science of the Total Environment.
DOE/EPRI. 2013. Electricity storage handbook in collaboration with NRECA. USA: Sandia National Laboratories and Electric Power Research Institute.
Droste-Franke B. 2015. Review of the need for storage capacity depending on the share of renewable energies (Chap. 6). In Electrochemical energy storage for renewable sources and grid balancing. Netherlands: Elsevier.
EC. 2017. Communication from the commission to the European Parliament on the 2017 list of critical raw materials for the EU. European Commission.
EIA. 2017. Wind turbines provide 8% of U.S. generating capacity, more than any other renewable source. U.S. Energy information administration.
EIA. 2019a. Table 7.2a Electricity net generation total (all sectors). U.S. Energy Information Administration.
EIA. 2019b. Table 6.7.B. Capacity factors for utility scale generators not primarily using fossil fuels. U.S. Energy Information Administration.
EWG. 2013. Fossil and nuclear fuels – the Supply Outlook. Energy Watch Group.
Ferroni, F., et al. 2016. Energy Return on Energy Invested (ERoEI) for photovoltaic solar systems in regions of moderate insolation. Energy Policy 94: 336–344
Flavelle, C., et al. 2019. U.S. Nuclear power plants weren’t built for climate change. Bloomberg.
Friedemann A. 2015. When trucks stop running: Energy and the future of transportation. Springer.
Frondel M, et al. 2006. Trends der angebots- und nachfragesituation bei mineralischen rohstoffen. Federal ministry of economics and energy.
GAO. 2010. Rare earth materials in the defense supply chain. U.S. Government Accountability Office.
Graedel TE, et al. 2015. On the materials basis of modern society. U.S. Proceedings of the National Academy of Sciences.
Hageluken C, et al. 2012. Precious Materials Handbook, Ch 1. Hanua-Wolfgang.
Hirsch H, et al. 2005. Nuclear reactor hazards ongoing dangers of operating nuclear technology in the 21st century. Amsterdam: Greenpeace International.
Hoffert MI, et al. 2002. Advanced technology paths to global climate stability: energy for a greenhouse planet. Science 298: 981-987.
HS. 2011. Dams and energy sectors interdependency study. U.S. Department of Energy and Homeland Security.
GTM. 2019. Why Flexible Gas Generation Must Be Part of Deep Decarbonization. Greentechmedia.
Hippel FN, et al. 2016. Reducing the danger from fires in spent fuel pools. Science & Global security 24: 141-173.
House 112-115. 2012. The EMP threat: Examining the consequences. U.S. House of representatives.
House 113-68. 2014. EMP threat to critical infrastructure. U.S. House of representatives.
Hovorka S. 2009. Characterization of bedded salt for storage caverns: case study from the Midland Basin. Tx: Texas Bureau of Economic Geology.
HS. 2011. Dams and energy sectors interdependency study. U.S. Department of Energy and Homeland Security.
IEA. 2017. Bioenergy’s role in balancing the electricity grid and providing storage options – an EU perspective. International Energy Agency.
Kerr, R. A. March 2, 2012. Is the World Tottering on the Precipice of Peak Gold? Science 335: 1038-1039.
Kerr, RA. February 14, 2014. The Coming Copper Peak. Science 343:722-724.
Klein G, et al. 2005. California’s water-energy relationship. California Energy Commission.
Lyman E, et al. 2017. Nuclear safety regulation in the post-Fukushima era. Science 356: 808-809.
MacFarlane D. 2018. The Southwest might be in one of the worst mega-droughts in history. Weather.com
Mackay M. 29 Dec 2012. Wind turbines’ lifespan far shorter than believed, study suggests. The Courier.
Makansi J. 2007. Lights Out: The Electricity Crisis, the Global Economy, and what it means to you. Wiley.
Mendick R. 30 Dec 2012. Wind Farm Turbines wear sooner than expected. The Telegraph.
Michaelides EE. 2012. Alternative Energy Sources. Springer.
MISO. 2018. Planning year 2019-2020. Wind & solar capacity credit. Midcontinent Independent System Operator.
Moyer M. 2010. Fusion’s false dawn. Scientific American.
Munson R. 2008. From Edison to Enron: The business of power & what it means for the future of electricity. Praeger
Navigant. 2013. U.S. Offshore Wind Manufacturing and Supply Chain Development. U.S. Department of Energy.
NRC. 2008. Minerals, critical minerals, and the U.S. economy Chapter 2. National Academies Press.
NRC. 2013. An Assessment of the Prospects for Inertial Fusion Energy. National Research Council.
NREL. 1983. Wind Energy Resource Atlas of the United States. National Renewable Energy Laboratory.
NREL. 1991. U.S. Solar Radiation Resource Maps: Atlas for the solar radiation data manual for flat plate and concentrating collectors. National Renewable Energy Laboratory.
NREL. 2010. Assessment of offshore wind energy resources for the United States. National Renewable Energy Laboratory, U.S. Department of Energy.
NREL. 2012. Geothermal power and interconnection: The economics of getting to market. National Renewable Energy Laboratory.
NREL. 2013a. Beyond renewable portfolio standards: An assessment of regional supply and demand conditions affecting the future of renewable energy in the west. Golden: National Renewable Energy Laboratory.
NREL. 2013b. Concentrating solar power projects. Colorado: National Renewable Energy Laboratory.
Perlman D. 2014. Livermore Lab’s fusion energy tests get closer to ‘ignition’. San Francisco Chronicle.
Pitron G. 2020. The Rare Metals War: The Dark Side of Clean Energy and Digital Technologies. Scribe US.
PU. Princeton University. 2017. US nuclear regulators greatly underestimate potential for nuclear disaster, researchers say. Phys.org.
Roberts D. 2019. These huge new wind turbines are a marvel. They’re also the future. Vox.
SBC. 2013. Electricity storage. SBC Energy Institute.
SBC. 2014. Hydrogen-based energy conversion. California: SBC Energy Institute.
Scrosati B., et al. 2013. Lithium batteries. Advanced technologies and applications. Wiley.
Shaibani M. 2020. Solar panel recycling: turning ticking time bombs into opportunities. PV magazine.
Stella C. 2019. Unfurling the waste problem caused by wind energy. National Public Radio.
St. John J. 2020. Transmission Emerging as major Stumbling Block for State Renewable Targets. Greentechmedia
Sverdrup HU, et al. 2019. Assessing the long-term global sustainability of the production and supply for stainless steel. Biophysical economics and resource quality.
Temple J (2018) The $2.5 trillion reason we can’t rely on batteries to clean up the grid. Fluctuating solar and wind power require lots of energy storage, and lithium-ion batteries seem like the obvious choice—but they are far too expensive to play a major role. MIT Technology Review.
TWC. Energy use from mining. The World Counts.
UNEP. 2011. Recycling rates of metals. United Nations Environmental Programme.
Vazquez S, et al. 2010. Energy storage systems for transport and grid applications. IEEE Transactions on Industrial Electronics 57(12): 3884.
Veronese K. 2015. The high-stakes race to satisfy our need for the scarcest metals on earth. Prometheus books.
Vikström H. et al. 2013. Lithium availability and future production outlooks. Applied Energy, 110: 252-266.
Wolfson R. 1993. Nuclear choices: a citizen’s guide to nuclear technology. MIT Press.
5 Responses to Renewables: not enough minerals, energy or time and mining is destructive