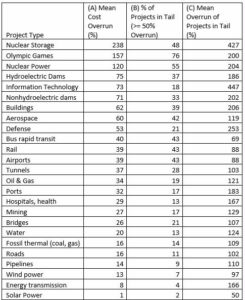
Megaprojects over $1 billion in order of likelihood to go over budget and timeline
Preface. This is a book review of Flyvbjerg et al “How Big Things Get Done: The Surprising Factors That Determine the Fate of Every Project, from Home Renovations to Space Exploration and Everything In Between.”
There are many reasons why big projects go over their budget, timeline, and generate fewer benefits than promised. Only 8.5% of projects hit the mark on both cost and time. And a minuscule 0.5% nail cost, time, and benefits, and were completed on time, on budget, and delivered promised benefits. Or to put that another way, 91.5% of projects go over budget, over schedule, or both. And 99.5% of projects go over budget, over schedule, under benefits, or some combination of these.
This is a huge problem for renewables. Nuclear above all, the #1 type of 25 projects that take much longer and cost more than predicted. Not just the existing light water reactors (LWR) either, but the new-fangled “advanced” small nuclear reactors (hardly “advanced” since many have been around for 70 years and have yet to succeed).
Fusion, Hydropower / dams, the California electric rail, and pumped hydropower are also expensive and complex. So are utility scale batteries, the electric grid expansion to charge batteries, heat homes and do everything else for that matter, and the large solar and wind farms to generate the electricity. Hydrogen infrastructure. Compressed Air energy systems to store electricity.
If peak oil was in 2018, then we don’t have the luxury of time. To electrify the grid requires tripling the amount of power we already generate. Probably 10 times more given that electricity will do EVERYTHING. Or 20 times more since months of electricity need to be stored to cope with seasonal wind and solar.
Other causes of project failures are a lack of experience, not being able to borrow the billions to construct a project, getting permits and fighting NIMBYism, being overly optimistic, politics, Black Swans (the longer a project takes, the more likely a black swan will occur), underestimating the cost in order to get funding, not enough planning, poor management, supply chain failures, bad weather, natural disaster (earthquake etc), and much more.
Alice Friedemann www.energyskeptic.com Author of Life After Fossil Fuels: A Reality Check on Alternative Energy; When Trucks Stop Running: Energy and the Future of Transportation”, Barriers to Making Algal Biofuels, & “Crunch! Whole Grain Artisan Chips and Crackers”. Women in ecology Podcasts: WGBH, Financial Sense, Jore, Planet: Critical, Crazy Town, Collapse Chronicles, Derrick Jensen, Practical Prepping, Kunstler 253 &278, Peak Prosperity, Index of best energyskeptic posts
***
Flyvbjerg B (2023) How Big Things Get Done: The Surprising Factors That Determine the Fate of Every Project, from Home Renovations to Space Exploration and Everything In Between. Crown Currency.
Nuclear power plants are one of the worst-performing project types in my database, with an average cost overrun of 120% in real terms and schedules running 65% longer than planned. Even worse, they are at risk of fat-tail extremes for both cost and schedule, meaning they may go 20 or 30% over budget. Or 200 or 300%. Or 500%. Or more. There is almost no limit to how bad things can get.
Nuclear power (from table below)
Project Type |
(A) Mean Cost Overrun (%)* |
(B) % of Projects in Tail (>= 50% Overrun) |
(C) Mean Overrun of Projects in Tail (%) |
Nuclear Power |
120 |
55 |
204 |
You build only one thing. By definition, that thing is one of a kind. To put that in the language of tailors, it is bespoke: no standard parts, no commercial off-the-shelf products, no simple repetition of what was done last time. And that translates into slow and complex. Nuclear power plants, for one, are the products of a staggering number of bespoke parts and systems that must all work, and work together, for the plant as a whole to work.
You can’t build a nuclear power plant quickly, run it for a while, see what works and what doesn’t, then change the design to incorporate the lessons learned. It’s too expensive and dangerous. That means that experimentation is out. You have no choice but to get it right the first time.
Second, there’s a problem with experience. If you are building a nuclear power plant, chances are that you haven’t done much of that before for the simple reason that few have been built and each takes many years to complete, so opportunities to develop experience are scarce. Yet with no experimentation and little experience, you still have to get it exactly right the first time. That’s difficult, if not impossible.
Even if you do have some experience building nuclear power plants, you probably won’t have experience building this particular nuclear plant because, with few exceptions, each plant is specifically designed for a specific site, with technology that changes over time.
The new “advanced” small nuclear reactors (SMR) are being sold as modular and easy to repeat. NOT TRUE. There are dozens of designs, many of them never succeeded, and it will take a long time for the NRC to get up to speed on so many options.
Here’s an example of an SMR that didn’t work out. In 1983, the government of Japan launched a new project that was as promising as it was enormous. Its name was Monju, meaning “wisdom.” When completed, Monju would be both a nuclear power plant churning out electricity for consumers and a fast-breeder reactor, a new type of nuclear plant that would produce fuel for the nuclear industry. For a nation long threatened by energy insecurity, Monju was designed to deliver a better future. Construction started in 1986. It finished almost a decade later, in 1995. But a fire immediately shut down the facility. An attempt to cover up the accident became a political scandal that kept the plant closed for years.
In 2000, the Japan Atomic Energy Agency announced that the plant could restart. Japan’s supreme court finally authorized the restart in 2005. Operations were scheduled to begin in 2008, but they were postponed until 2009. Test runs started in 2010, with full operations scheduled to commence, for the first time, in 2013. But in May 2013, maintenance flaws were discovered on some 14,000 components of the plant, including critical safety equipment. The restart was halted. Further violations of safety protocols were uncovered. Japan’s Nuclear Regulation Authority declared Monju’s operator to be unqualified. At that point, the government had spent $12 billion and the estimated cost of finally restarting Monju and operating it for ten years was another $6 billion—at a time when the 2011 Fukushima disaster had turned popular opinion against nuclear power. In 2016, it announced that Monju would be permanently closed.
Decommissioning Monju is expected to take another 30 years and cost a further $3.4 billion. If that forecast proves more accurate than the rest, the project will have taken 60 years, cost more than $15 billion, and produced zero electricity.[5]
Lacking experimentation and experience, what you learn as you proceed is that the project is more difficult and costly than you expected
Obstacles that were unknown are encountered. Solutions thought to work don’t. And you cannot make up for it by tinkering or starting over with revised plans. Operations experts call this “negative learning”: The more you learn, the more difficult and costly it gets.
Third, there’s the financial strain. A nuclear power plant must be completely finished before it can generate any electricity. So all the money that is poured into the plant produces nothing for the entire time it takes you to get to the ribbon-cutting ceremony—which, given the bespokeness, the complexity, the lack of experimentation, your lack of experience, the negative learning, and the need to get everything right the first time, will probably be a very long time. All this is reflected in the dreadful performance data for nuclear power plants.
Finally, don’t forget black swans. All projects are vulnerable to unpredictable shocks, with their vulnerability growing as time passes. So the fact that the delivery of your one huge thing will take a very long time means that it is at high risk of being walloped by something you cannot possibly anticipate. That’s exactly what happened to Monju. More than a quarter century after the project was launched, when the plant was still not ready to go, an earthquake caused a tsunami that struck the nuclear plant at Fukushima, producing the disaster that turned public opinion against nuclear power and finally convinced the Japanese government to pull the plug on Monju.…
It’s important to note that modularity is a matter of degree. The Empire State Building wasn’t modular to the extent that a Lego model of the Empire State Building is modular, but its floors were designed to be as similar as possible, with many being identical, which meant that workers often repeated work, which helped them learn and work faster. Similarly, the construction of the Pentagon was accelerated by keeping the five sides of the building identical. Following this line of reasoning, I advised a company building a large nuclear power plant to duplicate exactly what it had done in building a recent plant, not because the earlier plant had been a great success but because even that degree of repetition would boost them up the learning curve.
California High-Speed Rail
In 2019, California’s governor announced that the state would complete only part of the route: the 171-mile section between the towns of Merced and Bakersfield, in California’s Central Valley, at an estimated cost of $23 billion. But when that inland section is completed, the project will stop. It will be up to some future governor to decide whether to launch the project again and, if so, figure out how to get the roughly $80 billion—or whatever the number will be by then—to extend the tracks and finally connect Los Angeles and San Francisco.
Consider that the cost of the line between only Merced and Bakersfield is the same as or more than the annual gross domestic product of Honduras, Iceland, and about a hundred other countries. And that money will build the most sophisticated rail line in North America between two towns most people outside California have never heard of. It will be—as critics put it—the “bullet train to nowhere.
Look at California High-Speed Rail. When it was approved by voters and construction started, there were lots of documents and numbers that may have superficially resembled a plan. But there was no carefully detailed, deeply researched, and thoroughly tested program, which is to say that there was no real plan.
When the project got under way it could at best be described as a “vision” or an “aspiration.
It’s a safe bet that when California governor Gavin Newsom decided not to scrap the California High-Speed Rail project, only curtail it, he and his aides were thinking very carefully about how sunk costs would weigh on the public mind, and they knew that scrapping the project would be interpreted by the public as “throwing away” the billions of dollars already spent.
I expect that California’s “bullet train to nowhere” will one day be the standard textbook illustration of the sunk-cost fallacy and escalation of commitment
by its impressive detail, it may give the false idea that the overall plan is stronger than it is, like a beautiful facade with no structure behind it. Governments and bureaucratic corporations are good at churning out this sort of analysis. It is a major reason why the California High-Speed Rail project was able to spend more than a decade “in planning” before construction began, producing impressive quantities of paper and numbers without delivering a plan worthy of the name.
One big one is politics. The ambition to be the first, the biggest, the tallest, or some other superlative is another.
Politicians everywhere know that awarding contracts to domestic companies is a good way to make influential friends and win public support with promises of jobs, even if the domestic company will not perform as well as its foreign competitor because it is less experienced. When this happens—and it happens routinely—those responsible put other interests ahead of achieving the project’s goal.
Big projects involve big money and big self-interest. And since “who gets what” is the core of politics, there is politics in every big project, whether public or private.
This fact helps explain why the California High-Speed Rail project became such a mess. There is no real high-speed rail in the United States, which suggests how much experience US companies have building it. When California started to seriously consider this type of rail, foreign companies with lots of experience—notably SNCF, the French National Railway Company—set up offices in California in the hope of landing a sole-source contract or at least being a major partner in the project’s development. But the state decided not to go that way. Instead, it hired a large number of mostly inexperienced, mostly US contractors and oversaw them with managers who also had little or no experience with high-speed rail.[2
Ticket price estimates were provided in a range of scenarios, with a low of $68 and a high of $104. The total cost of the project was estimated at between $32.785 billion and $33.625 billion. See California High-Speed Rail Authority, Financial Plan (Sacramento: California High-Speed Rail Authority, 1999); California High-Speed Rail Authority, California High-Speed Train Business Plan (Sacramento: California High-Speed Rail Authority, 2008); Safe, Reliable High-Speed Passenger Train Bond Act for the 21st Century, AB-3034, 2008, https://leginfo.legislature.ca.gov/?faces/?billNavClient.xhtml?bill_id=200720080AB3034.
How do visions become plans that deliver successful projects? Not like this.
PSYCHOLOGY
One driver is psychology. In any big project—meaning a project that is considered big, complex, ambitious, and risky by those in charge—people think, make judgments, and make decisions. And where there are thinking, judgment, and decisions, psychology is at play; for instance, in the guise of optimism
Another driver is power. In any big project people and organizations compete for resources and jockey for position.
In every big project, there are blizzards of numbers generated at different stages by different parties. Finding the right ones—those that are valid and reliable—takes skill and work. Even trained scholars get it wrong.[4] And it doesn’t help that big projects involve money, reputations, and politics. Those who have much to lose will spin the numbers, so you cannot trust them. That’s not fraud. Or rather, it’s not usually fraud; it’s human nature. And with so many numbers to choose from, spinning is a lot easier than finding the truth.
This is a serious problem. Projects are promised to be completed by a certain time, at a certain cost, with certain benefits produced as a result—benefits being things such as revenues, savings, passengers moved, or megawatts of electricity generated. So how often do projects deliver as promised? That is the most straightforward question anyone could ask. But when I started to look around in the 1990s, I was stunned to discover that no one could answer it. The data simply hadn’t been collected and analyzed. That made no sense when trillions of dollars had been spent on the giant projects increasingly being called megaprojects—projects with budgets in excess of $1 billion.
Our database started with transportation projects: the Holland Tunnel in New York; the BART system in San Francisco; the Channel Tunnel in Europe; bridges, tunnels, highways, and railways built throughout the twentieth century. It took five years, but with my team I got 258 projects into the database, making it the biggest of its kind at the time.[5]
“Project estimates between 1910 and 1998 were short of the final costs an average of 28 percent,” according to The New York Times, summarizing our findings. “The biggest errors were in rail projects, which ran, on average, 45 percent over estimated costs [in inflation-adjusted dollars]. Bridges and tunnels were 34 percent over; roads, 20 percent. Nine of 10 estimates were low, the study said.”[7] The results for time and benefits were similarly bad.
Measured differently—from an earlier date and including inflation—the numbers are much worse.
I worked with McKinsey, and indeed we found that IT disasters were even worse than transportation disasters. We shifted our research to mega-events such as the Olympic Games and got the same result. Big dams? Same again. Rockets? Defense? Nuclear power? The same. Oil and gas projects? Mining? Same. Even something as common as building museums, concert halls, and skyscrapers fit the pattern. I was astonished.[10] And the problem wasn’t limited to any country or region; we found the same pattern all over the world.[11] The famously efficient Germans have some remarkable examples of bloat and waste, including Berlin’s new Brandenburg Airport, which was years delayed and billions of euros over budget, Even Switzerland, the nation of precise clocks and punctual trains, has its share of embarrassing projects; for instance, the Lötschberg Base Tunnel, which was completed late and with a cost overrun of 100 percent.
The pattern was so clear that I started calling it the “Iron Law of Megaprojects”: over budget, over time, under benefits, over and over again.[13]
the probability that any big project will blow its budget and schedule and deliver disappointing benefits is very high and very reliable.
The database that started with 258 projects now contains more than 16,000 projects from 20-plus different fields in 136 countries on all continents except Antarctica, and it continues to grow.
Only 8.5 percent of projects hit the mark on both cost and time. And a minuscule 0.5 percent nail cost, time, and benefits, and were completed on time, on budget, and delivered promised benefits
Or to put that another way, 91.5 percent of projects go over budget, over schedule, or both. And 99.5 percent of projects go over budget, over schedule, under benefits, or some combination of these.
you have assumed that if you are hit with a cost overrun, it will be somewhere around the mean—or 62 percent. Why did you assume that? Because it would be true if the cost overruns followed what statisticians call a “normal distribution.” That’s the famous bell curve.
But the “normal” distribution isn’t the only sort of distribution that exists. Other distributions that are called “fat-tailed” because, compared with normal distributions, they contain far more extreme outcomes in their tails. Wealth, for example, is fat-tailed. At the time of writing, the wealthiest person in the world is 3,134,707 times wealthier than the average person.
If human height followed the same distribution as human wealth, the tallest person in the world would not be 1.6 times taller than the average person; he would be 3,311 miles (5,329 kilometers) tall, meaning that his head would be thirteen times farther into outer space than the International Space Station. Another example of a fat tail distribution are the costs of natural disasters with rising severity
Are project outcomes distributed “normally,” or do they have fat tails? My database revealed that information technology projects have fat tails. To illustrate, 18% of IT projects have cost overruns above 50% in real terms. And for those projects the average overrun is 447%! That’s the average in the tail, meaning that many IT projects in the tail have even higher overruns than this. Information technology is truly fat-tailed!
So are nuclear storage projects. And the Olympic Games. And nuclear power plants. And big hydroelectric dams. As are airports, defense projects, big buildings, aerospace projects, tunnels, mining projects, high-speed rail, urban rail, conventional rail, bridges, oil projects, gas projects, and water projects. Most project types have fat tails. How “fat” their tails are—how many projects fall into the extremes and how extreme those extremes are—does vary. I’ve cited them in order, from fattest to least fat (but still fat)—or, if you prefer, from most at risk of terrifying overruns to less at risk (but still very much at risk).
Most project types are not only at risk of coming in late, going over budget, and generating fewer benefits than expected. They are at risk of going disastrously wrong. That means you may not wind up 10% over budget; you may go 100% over. Or 400%. Or worse. These are black swan outcomes, and the project types at risk of them are called “fat-tailed.” They include nuclear power plants, hydroelectric dams, information technology, tunnels, major buildings, aerospace, and many more.
The table shows cost overruns for 25 project types covering 16,000 plus projects. Overrun is measured as (a) mean cost overrun, (b) percentage of projects in the upper tail (defined as = 50 percent), and (c) mean overrun in the tail. Overrun is measured in real terms. The numbers in the table are base rates for cost risk in project management. For example, if you’re planning to host the Olympic Games, your base rate (expected value) for cost overrun will be 157 percent, with a 76 percent risk of ending up in the tail with an expected overrun of 200 percent and substantial further risk of overrun above this.
We see from the table that the base rates are very different for different project types for both mean risk and tail risk. The highest mean risk is found for nuclear storage, at 238%, while the lowest is found for solar power, at 1 percent. The highest risk for ending up in the tail is held by the Olympics, at 76 percent, while the highest mean overrun in the tail is found for IT projects, at 447 percent. Differences in base rates must be taken into account when planning and managing projects but often are not. Frequently, empirical base rates are not considered at all
Cost overrun was calculated not including inflation and baselined as late in the project cycle as possible, just before the go-ahead (final business case at final investment decision). This means that the numbers in the table are conservative. If inflation had been included and early business cases used as the baseline, cost overrun would be much higher, sometimes several times higher.
What do fat-tailed outcomes look like? Boston’s “Big Dig”—replacing an elevated highway with a tunnel, with construction started in 1991—put the city through the wringer for 16 years and cost more than triple what it was supposed to.
NASA’s James Webb Space Telescope, which is now almost a million miles from Earth, was forecast to take 12 years but required 19 to complete, while its final cost of $8.8 billion was an astronomical—forgive me—450% over budget.
My data show that smaller projects are susceptible to fat tails, too. Moreover, fat-tailed distributions, not normal distributions, are typical within complex systems, both natural and human, and we all live and work within increasingly complex systems, which means increasingly interdependent systems. Cities and towns are complex systems.
Markets are complex systems. Energy production and distribution are complex systems. Manufacturing and transportation are complex systems. Debt is a complex system. So are viruses. And climate change. And globalization. On and on the list goes. If your project depends on other people and many parts, it is all but certain that your project is embedded in complex systems.
That describes projects of all types and scales, all the way down to home renovations.
Nassim Nicholas Taleb famously dubbed low-probability, high-consequence events “black swans.” Disastrous project outcomes such as these can end careers, sink companies, and inflict a variety of other carnage. They definitely qualify as black swans.
1 |
Earthquakes |
On Richter scale |
2 |
Cybercrime |
Financial loss |
3 |
Wars |
# of battle deaths per capita of involved nations |
4 |
Pandemics |
Number of deaths |
5 |
IT procurement |
% size of cost overrun |
6 |
Floods |
volume of water |
7 |
Bankruptcies |
% of firms per year per industry |
8 |
Forest fires |
size of area affected |
9 |
Olympic Games |
% size of cost overrun |
10 |
Blackouts |
# of customers affected |
Major corporations on the hook for runaway projects may be able to keep things going by borrowing more and more money. Governments can also pile up debt. Or raise taxes.
Projects that fail tend to drag on, while those that succeed zip along and finish.
Think of the duration of a project as an open window. The longer the duration, the more open the window. The more open the window, the more opportunity for something to crash through and cause trouble, including a big, bad black swan. What could that black swan be? Almost anything. It could be something dramatic, like an election upset, a stock market collapse, or a pandemic.
Events such as these may be extremely unlikely on any given day, month, or year. But the more time that passes from the decision to do a project to its delivery, the greater their probability. The more time that passes from decision to delivery, the greater the probability of one or more of these events happening.
Gusts, at just the wrong moment, that pushed the bow of Ever Given, a giant container ship, into a bank of the Suez Canal. The ship got stuck and couldn’t be budged for six days, blocking the canal, halting hundreds of ships, freezing an estimated $10 billion in trade each day, and sending shocks rippling through global supply chains. The people and projects who suffered as a result of those supply-chain troubles may never have realized it, but the cause of their trouble was ultimately strong winds in a faraway desert.[23] A complex systems theorist might describe what happened by saying that the dynamic interdependencies among the parts of the system—the wind, the canal, the ship, and supply chains—created strong nonlinear responses and amplification.
Minor changes combined in a way to produce a disaster. In complex systems, that happens so often that the Yale sociologist Charles Perrow called such events “normal accidents.”[24] Growing complexity and interdependency may make such outcomes more likely in today’s world. From the dramatic to the mundane to the trivial, change can rattle or ruin a project—if it occurs during the window of time when the project is ongoing. Solution? Close the window by speeding up the project and bringing it to a conclusion faster.
BUT CONSTRUCTING TOO FAST IS A PROBLEM
When Møller asked the architect, Henning Larsen, how long it would take, Larsen said five years. “You’ll get four!” Møller curtly responded. But the cost of that haste was terrible, and not only in terms of cost overruns. Larsen was so appalled by the completed building that he wrote a whole book to clear his reputation
In 2021, after an overpass collapsed beneath a metro train in Mexico City, three independent investigations concluded that rushed, shoddy work was to blame.
The New York Times did its own investigation and concluded that the city’s insistence that construction be completed before the city’s powerful mayor was due to leave office had been a key contributing cause of the collapse. “The scramble led to a frenzied construction process that began before a master plan had been finalized and produced a metro line with defects from the start,” the Times concluded.
On project after project, rushed, superficial planning is followed by a quick start that makes everybody happy because shovels are in the ground. But inevitably, the project crashes into problems that were overlooked or not seriously analyzed and dealt with in planning. People run around trying to fix things. More stuff breaks. There is more running around.
PLANNING SHOULD BE SLOW
Most planning is done with computers, paper, and physical models, meaning that planning is relatively cheap and safe. Barring other time pressures, it’s fine for planning to be slow. Delivery is another matter. Delivery is when serious money is spent and the project becomes vulnerable as a consequence. Planning is a safe harbor. Delivery is venturing across the storm-tossed seas. This is a major reason why, at Pixar—the legendary studio that created Toy Story, Finding Nemo, The Incredibles, Soul, and so many other era-defining animated movies—“directors are allowed to spend years in the development phase of a movie. A Pixar movie usually goes through the cycle from script to audience feedback eight times. The amount of change between versions one and two “is usually huge,” said Docter. “Two to three is pretty big. And then, hopefully, as time goes on, there are enough elements that work that the changes become smaller and smaller.
Cultivating ideas and innovations takes time. Spotting the implications of different options and approaches takes more time. Puzzling through complex problems, coming up with solutions, and putting them to the test take still more time. Planning requires thinking—and creative, critical, careful thinking is slow.
SOMERVELL CHOSE HORRIBLE SPOT TO PUT THE PENTAGON ON. Why didn’t he realize that there was a much better site available before he sought and got approval for the original design? Why didn’t any of those who approved the plan spot the flaw? Because Somervell’s plan was so absurdly rushed and superficial that no one had even looked for other sites, much less considered their relative merits carefully. They had all treated the first suitable site identified as the only suitable site. Somervell fought back. He criticized the critics and insisted that only the Arlington Farm site was suitable. As he drove his staff even harder to start digging a hole. Critics urged the president to intervene. He did, telling Somervell to switch sites. Incredibly, Somervell still wouldn’t quit.
Somervell presented his plan to the secretary of war, a congressional subcommittee, and the White House cabinet, including the president. Each time, so few probing questions were asked that the blatant flaws in the plan were not revealed. And each time, the plan was quickly approved. Somervell’s superiors simply didn’t do their jobs. The fact that the flaw was eventually exposed and the plan stopped—in part because one of the determined critics of the plan happened to be the president’s uncle—only underscores their dereliction of duty and the arbitrariness of the decision.
Purposes and goals are not carefully considered. Alternatives are not explored. Difficulties and risks are not investigated. Solutions are not found. Instead, shallow analysis is followed by quick lock-in to a decision that sweeps aside all the other forms the project could take. “Lock-in,” as scholars refer to it, is the notion that although there may be alternatives, most people and organizations behave as if they have no choice but to push on. I call such premature lock-in the “commitment fallacy.
The only thing that is truly unusual about the Pentagon story is that a group of politically connected critics managed to expose the flaws in Somervell’s plan after it had been approved and get the project moved to another site—the one where the Pentagon is today.
One is what I call “strategic misrepresentation,” the tendency to deliberately and systematically distort or misstate information for strategic purposes. If you want to win a contract or get a project approved, superficial planning is handy because it glosses over major challenges, which keeps the estimated cost and time down, which wins contracts and gets projects approved.
But as certain as the law of gravity, challenges ignored during planning will eventually boomerang back as delays and cost overruns during delivery. By then the project will be too far along to turn back. Getting to that point of no return is the real goal of strategic misrepresentation. It is politics, resulting in failure by design.
A second is psychology, and politics for Kahneman. Which factor is most important depends on the character of decisions and projects. In Kahneman’s laboratory experiments, the stakes are low. There is typically no jockeying for position, no competition for scarce resources, no powerful individuals or organizations, no politics of any kind. The closer a project is to that situation, the more individual psychology will dominate. As projects get bigger and decisions more consequential, the influence of money and power grows. Powerful individuals and organizations make the decisions,
We are a deeply optimistic species. That makes us an overconfident species. The large majority of car drivers say that their driving skills are above average. Unchecked, optimism leads to unrealistic forecasts, poorly defined goals, better options ignored, problems not spotted and dealt with, and no contingencies to counteract the inevitable surprises. Yet, as we will see in later chapters, optimism routinely displaces hard-nosed analysis in big projects, as in so much else people do.
One of the basic insights of modern psychology is that quick and intuitive “snap judgments” are the default operating system of human decision making—“System One,” to use the term coined by the psychologists Keith Stanovich and Richard West and made famous by Kahneman.
Conscious reasoning is a different system: System Two. A key difference between Systems One and Two is speed. To generate snap judgments, the brain can’t be overly demanding about information. Instead, it proceeds on the basis of what Kahneman calls “WYSIATI” (What You See Is All There Is), meaning an assumption that whatever information we have on hand is all the information available to make the decision.
When we have a strong intuitive judgment we seldom subject it to slow, careful, critical scrutiny. We just go with it. Emotions such as anger, fear, love, or sadness may inspire rash conclusions as well. We all know—at least when we’re thinking coolly—that strong emotions are not necessarily logical or supported by evidence and are therefore an unreliable basis for judgment.
But the intuitive judgments generated by System One are not experienced as emotions. They simply “feel” true. With the truth in hand, it seems perfectly reasonable to act on it. This is what makes optimism bias so potent. Small-business owners who are sure they will avoid the fate of most small-business owners
it’s important to recognize that quick, intuitive judgment often works remarkably well. That’s why it is our default. This is typical of the planning of big projects. It’s just not suitable for the sort of quick-and-intuitive decision making that comes naturally to us. But all too often we apply it anyway—because it comes naturally to us.
Forty years ago, Kahneman and Tversky showed that people commonly underestimate the time required to complete tasks even when there is information available that suggests the estimate is unreasonable. They called this the “planning fallacy,
The physicist and writer Douglas Hofstadter mockingly dubbed it “Hofstadter’s Law”: “It always takes longer than you expect, even when you take into account Hofstadter’s Law.
You expect to get downtown on a Saturday night within twenty minutes, but it takes 40 minutes instead and now you’re late—just like last time. Incredibly, when people said there was a 99% probability that they would be done—that it was virtually certain—only 45% actually finished by that time.
For us to be so consistently wrong, we must consistently ignore experience. And we do, for various reasons. When we think of the future, the past may simply not come to mind and we may not think to dig it up because it’s the present and future we are interested in. If it does surface, we may think “This time is different” and dismiss it. Or we may just be a little lazy and prefer not to bother, a preference well documented in Kahneman’s work. We all do this. Think of taking work home on the weekend. It’s a safe bet that what you will get done is less than what you planned.
Using a best-case scenario as the basis for an estimate is a really bad idea because the best case is seldom the most likely way the future will unfold. It’s often not even probable. There is an almost infinite number of things that could pop up during the weekend and eat into your work time: illness, an accident, insomnia, a phone call from an old friend, a family emergency, broken plumbing, and on down the list.
If this sort of casual forecasting seems miles removed from the cost and time estimates of big projects, think again. It’s common for such estimates to be made by breaking a project down into tasks, estimating the time and cost of each task, then adding up the totals.
Experience—in the shape of past outcomes of similar projects—is often ignored, and little or no careful consideration is given to the many ways the forecasts could be knocked off-kilter. These are effectively forecasts based on best-case scenarios
“Speed matters in business,” notes one of Amazon’s famous leadership principles, written by Jeff Bezos.
Notice, however, that Bezos carefully limited the bias for action to decisions that are “reversible. It is also inapplicable to many decisions on big projects because they are so difficult or expensive to reverse
When this bias for action is generalized into the culture of an organization, the reversibility caveat is usually lost. What’s left is a slogan—“Just do it! Managers feel more productive executing tasks than planning them,
People in power, which includes executives deciding about big projects, prefer to go with the quick flow of availability bias, as opposed to the slow effort of planning. Executives everywhere will recognize that attitude. It emerges from a desire to get going on a project, to see work happening, to have tangible evidence of progress.
Planning is working on the project. Progress in planning is progress on the project, often the most cost-effective progress you can achieve.
THE POLITICS OF OVERESTIMATION
“In France, there is often a theoretical budget that is given because it is the sum that politically has been released to do something. In three out of four cases this sum does not correspond to anything in technical terms. This is a budget that was made because it could be accepted politically. The real price comes later. The politicians make the real price public where they want and when they want.” That’s a long way of saying that estimates aren’t intended to be accurate; they are intended to sell the project. In a word, they are lies
“News that the Transbay Terminal is something like $300 million over budget should not come as a shock to anyone,” wrote Willie Brown, a former San Francisco mayor and California state assemblyman. “We always knew the initial estimate was under the real cost. Just like we never had a real cost for the Central Subway or the Bay Bridge or any other massive construction project. So get off it. In the world of civic projects, the first budget is really just a down payment. If people knew the real cost from the start, nothing would ever be approved”. With contracts signed, the next step is to get shovels in the ground. Fast. “The idea is to get going,” concluded Willie Brown. “Start digging a hole and make it so big, there’s no alternative to coming up with the money to fill it in.”
Needless to say, Brown was retired from politics when he wrote that.
The editor of a major US architecture and design magazine even rejected an article I pitched about strategic misrepresentation on the grounds that lying about projects is so routine that his readers take it for granted, and thus the article wouldn’t be newsworthy.
Rushed, superficial planning is no problem for the creation of a lowball estimate. In fact, it can be enormously helpful. Problems and challenges overlooked are problems and challenges that won’t increase the estimate.
It also helps to express ironclad confidence in estimates, as Montreal mayor Jean Drapeau did when he promised that the 1976 Olympic Games would cost no more than what was budgeted. Future embarrassment awaits when you talk like that. But that’s for later. After you get what you want. And maybe after you’ve retired.
Elia Kazan—then retired, naturally—when he explained how he had gotten Columbia Pictures to fund a movie he wanted to make in the late 1940s. “Get the work rolling, involve actors contractually, build sets, collect props and costumes, expose negative, and so get the studio in deep. Once money in some significant amount had been spent, it would be difficult for Harry [Cohn, president of Columbia Pictures] to do anything except scream and holler.
Today, Heaven’s Gate is famous in Hollywood, but not in a good way. It ultimately cost five times the initial estimate and opened a year late. The critical reaction was so savage that Cimino withdrew the film, reedited it, and released it again six months later. It bombed at the box office. And United Artists was wiped out.
One element that is central to any account is the “sunk cost fallacy.” Money, time, and effort previously spent to advance a project are lost in the past. You can’t get them back. They are “sunk.” Logically, when you decide whether to pour more resources into a project, you should consider only whether doing so makes sense now. Sunk costs should not be a factor in your thinking—but they probably will be because most people find it incredibly hard to push them out of their minds. And so people commonly “throw good money after bad,” to use the old phrase.
On the day of the game, there is a big snowstorm. The higher the price the friends paid for the tickets—their sunk costs—the more likely they are to brave the blizzard and attempt driving to the game, investing more time, money, and risk. “Driving into the blizzard” is particularly common in politics. Sometimes that’s because politicians themselves fall for it. But even politicians who know better understand that the public is likely to be swayed by sunk costs, so sticking with a fallacy is politically safer than making a logical decision.
We should be careful not to see psychology and politics as separate forces; they can be mutually reinforcing and typically are in big projects. When they align in favor of a superficial plan and a quick start, that’s probably what will occur—with predictable consequences.
Good planning explores, imagines, analyzes, tests, and iterates. That takes time. Thus, slow is a consequence of doing planning right, not a cause. The cause of good planning is the range and depth of the questions it asks and the imagination and the rigor of the answers it delivers. Notice that I put “questions” before “answers.” It’s self-evident that questions come before answers. Or rather, it should be self-evident.
Projects routinely start with answers, not questions. What Gehry means by questioning isn’t doubting or criticizing, much less attacking or tearing down. He means asking questions with an open-minded desire to learn. It is, in a word, exploration. “You’re being curious,” he says. That’s the opposite of the natural inclination to think that What You See Is All There Is (WYSIATI). The whole conversation starts with a simple question: “Why are you doing this project?” Few projects start this way. All should. As brutal as the experience was, the process of building the Disney Concert Hall taught Gehry a host of lessons that he used in building the Guggenheim Bilbao and has used in projects ever since. Who has power, and who doesn’t? What are the interests and agendas at work? How can you bring on board those you need and keep them there? How do you maintain control of your design? These questions are as important as aesthetics and engineering to the success of a project.
Bezos noted that when a project is successfully completed and it’s ready to be publicly announced, the conventional last step is to have the communications department write two documents. One is a very short press release (PR) that summarizes what the new product or service is and why it is valuable for customers. The other is a “frequently asked questions” (FAQ) document. To pitch a new project at Amazon, you must first write a PR and FAQ. The language of both documents must be plain. With language like that, flaws can’t be hidden behind jargon, slogans, or technical terms. Projects are pitched in a one-hour meeting with top executives. Amazon forbids PowerPoint presentations and all the usual tools of the corporate world, so copies of the PR/FAQ are handed around the table and everyone reads it, slowly and carefully, in silence. Then they share their initial thoughts, with the most senior people speaking last to avoid prematurely influencing others. Finally, the writer goes through the documents line by line, with anyone free to speak up at any time. “This discussion of the details is the critical part of the meeting, The writer of the PR/FAQ then takes the feedback into account, writes another draft, and brings it back to the group. The same process unfolds again. And again. And again. It ensures that the concept that finally emerges is seen with equal clarity in the minds of everyone
But no process is foolproof. When Jeff Bezos dreamed up the idea of a 3D phone display that would allow control by gestures in the air (the hand-waving again!), he fell in love with the concept. Later, he effectively co-authored the PR/FAQ that would launch the Amazon Fire Phone project. When the Fire Phone was released in June 2014 at a price of $200, it didn’t sell. The price was cut in half. Then the phone was free. Amazon couldn’t give it away. A year later, it was discontinued and hundreds of millions of dollars were written off. “It failed for all the reasons we said it was going to fail—that’s the crazy thing about it,” said a software engineer. Asking “Why?” can work only where people feel free to speak their minds and the decision makers really listen.
Caro is the greatest living American biographer, famous for long, deeply researched, massively complex books. “What is this book about?,” he asks himself. “What is its point?” He forces himself to “boil the book down to three paragraphs, or two, or one. These paragraphs express the narrative theme with perfect simplicity. But don’t mistake simple for easy. Caro writes a draft and throws it out. Then another. And another, in seemingly endless iterations. This can go on for weeks as he compares his summary with his voluminous research. “The whole time, I’m saying to myself, ‘no, that’s not exactly what you’re trying to do in this book.’ When he finally has his precious paragraphs, he pins them to the wall behind his desk, where it is literally impossible for him to lose sight of his goal.
The construction of the Sydney Opera House was an outright fiasco. Setbacks piled up. Costs exploded. Scheduled to take five years to build, it took fourteen. The final bill was 1,400 percent over the estimate, one of the largest cost overruns for a building in history. A bad plan is one that applies neither experimentation nor experience. The plan for the Sydney Opera House was very bad. Utzon’s entry was so sparse that it didn’t even satisfy all the technical requirements set by the organizers, but his simple sketches were indisputably brilliant—perhaps too brilliant. They mesmerized the jury and swept objections aside. The principal mystery lay in the curved shells at the heart of Utzon’s vision. They were beautiful on two-dimensional paper, but what three-dimensional constructs would enable them to stand? What materials would they be made of? How would they be built? None of that had been figured out. Utzon hadn’t even consulted engineers.
In bad planning, it is routine to leave problems, challenges, and unknowns to be figured out later. In many projects, the problem is never solved.
We all know that experience is valuable.
All else being equal, an experienced carpenter is a better carpenter than an inexperienced one. It should also be obvious that in the planning and delivery of big projects experience should be maximized whenever possible—by hiring the experienced carpenter, for example. It shouldn’t be necessary to say that. But it is necessary to say it—loudly and insistently—because, as we shall see, big projects routinely do not make maximum use of experience. In fact, experience is often aggressively marginalized. When the Canadian government decided it wanted to buy two icebreakers, it didn’t buy them from manufacturers in other countries that were more experienced. Rather than give the contracts to one company so that it could build one ship, learn from the experience, and deliver the second ship more efficiently, it gave one contract to one company and the other to another company. So why do it? One company is in a politically important region in Quebec, the other in a politically important region in British Columbia.
The desire to do what has never been done before can be admirable, for sure. But it can also be deeply problematic. First-mover advantage is greatly overstated. In a watershed study, researchers compared the fates of “pioneer” companies that had been the first to exploit a market and “settlers” that had followed the pioneers into the market. Drawing on data from five hundred brands in fifty product categories, they found that almost half of pioneers failed, compared to 8 percent of settlers. The surviving pioneers took 10 percent of their market, on average, compared to 28 percent for settlers.
Consider Seattle’s State Route 99 tunnel. A decade ago, when Seattle announced that it would bore a tunnel under its waterfront to replace a highway on the surface, it was far from a first, so there was plenty of relevant experience to draw on. But Seattle decided that its tunnel would be the biggest of its kind in the world, with enough room for two decks, each with two lanes of traffic. But to bore the world’s biggest tunnel, you need the world’s biggest boring machine. Such a machine, by definition, hasn’t been built and used before. It would be the first. That cost $80 million, which is more than double the price of a standard boring machine. After boring a thousand feet of a tunnel that would be nine thousand feet long, the borer broke down and became the world’s biggest cork in a bottle. Extracting it from the tunnel, repairing it, and getting it back to work took two years and cost another $143 million.
Project planners should prefer highly experienced technology for the same reason house builders should prefer highly experienced carpenters. But we often don’t see technology this way. Too often, we assume that newer is better.
Olympic Games. Since 1960, the total cost of hosting the Games—six weeks of competitions, including the Paralympics, held once every four years—has grown spectacularly and is now in the tens of billions of dollars. Every Olympic Games since 1960 for which data are available, summer and winter, has gone over budget. The average overrun is 157 percent in real terms. Only nuclear waste storage has higher cost overruns of the twenty-plus project categories my team and I study. There is no permanent host for the Games. Instead, the International Olympic Committee (IOC) calls on cities to bid for each Games. The IOC prefers to move the Games from region to region, continent to continent, because that’s an excellent way to promote the Olympic brand and therefore serves the interests of the IOC—which is to say, it is excellent politics.
Testing is all the more critical for those very rare big projects—such as finding solutions to the climate crisis, getting people to Mars, or permanently storing nuclear waste—that must do what has never been done before because that is the heart of the project. They start with a deep deficit of experience.
How did MTR know that its delivery was failing? By the schedule and budget slipping. But slippage was measured against MTR’s forecasts of how long and how costly the various stages of the project would be. If those forecasts were fundamentally unrealistic, a team expected to meet them would fail no matter what they did. The delivery would be doomed before it started. That should be obvious. But when things go wrong and people get desperate, the obvious is often overlooked,
Many small probabilities added together equal a large probability that at least some of those nasty surprises will actually come to pass. Your forecast did not account for that. That means your forecast, which seemed perfectly reasonable and reliable, was actually a highly unrealistic things-will-go-according-to-plan best-case scenario,
And things almost never go according to plan. On big projects, they don’t even come close.
You may think, as most people do, that the solution is to look more closely at your kitchen renovation, identify all the things that could possibly go wrong, and work them into your forecast. It’s not. Spotting ways that things can go wrong is important because it enables you to reduce or eliminate risks or mitigate them, as I’ll discuss below. But it won’t get you the foolproof forecast you want. No matter how many risks you can identify, there are always many more that you can’t. They are the “unknown unknowns. You just need to start over with a different perspective: See your project as one in a class of similar projects already done, as “one of those.” Use data from that class—about cost, time, benefits, or whatever else you want to forecast—as your anchor. Then adjust up or down, if necessary, to reflect how your specific project differs from the mean in the class. That’s it. It couldn’t be simpler.
shoddy forecasting is the bread and butter of countless corporations. They do not want the people who authorize projects and pay the bills to have a more accurate picture of what projects will cost and how long they will take.
Kahneman writes about a time when he and some colleagues set out to compose a textbook together. They all agreed that it would take roughly two years. But when Kahneman asked the only member of the group with considerable experience in producing textbooks how long it usually takes, that expert said he couldn’t recall any project taking less than seven years. The textbook was ultimately finished eight years later.
In the kitchen renovation example, I took for granted that you have data on kitchen renovations that will allow you to calculate the average cost. But you probably don’t. And you’ll struggle to find them. The same goes for your kitchen renovation. Look around for others who have done a kitchen renovation in, say, the past five to ten years. Ask friends, family, co-workers. Kitchen renovations are common, so let’s say you come up with fifteen such projects. Get the total cost of each, add them, divide by fifteen. That’s your anchor. Even simpler and even more accurate, you could get the percentage cost overrun for each renovation
In 2004, I got a call from Anders Bergendahl, the Swedish official in charge of decommissioning nuclear power plants. He needed a reliable estimate of how much it would cost to decommission Sweden’s fleet of nuclear power plants, which would take decades, and safely store nuclear waste, which would last for centuries. Sweden’s nuclear industry would be asked to pay into a fund to cover those costs, so the government needed to know how much the industry should pay. Sweden would be the first country to carry out a planned decommissioning of a fleet of reactors. “I can’t help,” I said.
But he had noticed a strange thing when he had compared the consultants’ report with an academic book in which my team and I had documented the cost risk for transportation infrastructure such as roads, bridges, and rail lines. According to our book, the cost risk was higher for that very ordinary sort of infrastructure.
only a minority of the many project types in my database are “normally” distributed. The rest—from the Olympic Games to IT projects to nuclear power plants and big dams—have more extreme outcomes in the tails of their distributions. With these fat-tailed distributions, the mean is not representative of the distribution and therefore is not a good estimator for forecasts. What does this mean in practice? Ideally, you’d always want to know whether you’re facing a fat-tailed distribution or not.
My team and I were asked to do just that for High Speed 2, or HS2, a $100 billion–plus high-speed rail line that will run from London to northern England, if and when it is completed. Using our database, we first explored the cost distribution of comparable high-speed rail projects around the world. Sure enough, the distribution had a fat tail. High-speed rail is risky business, as we saw in Hong Kong. So we zeroed in on the projects in the tail and investigated what exactly had made each project blow up. The answers were surprisingly simple. The causes had not been “catastrophic” risks such as terrorism, strike actions, or other surprises; they had been standard risks that every project already has on its risk register. We identified roughly a dozen of those and found that projects were undone by the compound effects of these on a project already under stress. We found that projects seldom nosedive for a single reason.
One of the most common sources of trouble for high-speed rail was archaeology. In many parts of the world, and certainly in England, construction projects are built on layers of history. The moment a project starts digging, there’s a good chance it will uncover relics of the past.
We also found that early delays in procurement and political decisions correlated with black swan blowouts in the HS2 reference class. Interestingly, early delays are not seen as a big deal by most project leaders. They figure they have time to catch up. But it’s dead wrong. Early delays cause chain reactions throughout the delivery process. The later a delay comes, the less remaining work there is do.
We had ten more items on the list of causes of high-speed rail black swans, including late design changes, geological risks, contractor bankruptcy, fraud, and budget cuts.
Projects that run into trouble and end in miserable failure are soon forgotten because most people aren’t interested in miserable failures; projects that run into trouble but persevere and become smash successes are remembered and celebrated.
Someone could note, for example, that Steve Jobs, Bill Gates, and Mark Zuckerberg all dropped out of university and conclude that a key to success in information technology is to leave school. What’s missing, and what makes that odd conclusion possible, are the dropouts who went nowhere in information technology and are ignored. That’s survivorship bias.
I know this conclusion is not emotionally satisfying. How could it be? The rare exceptions Hirschman incorrectly thought are typical are, almost by definition, fantastic projects that make irresistible stories. They follow the perfect Hero’s Journey, with a narrative arc from great promise to near ruin to an even greater accomplishment and celebration. We seem hardwired to love such stories. We crave them in all cultures and times. So there will always be authors telling those stories. Like Hirschman. Or Malcolm Gladwell.
Then there’s the Hoover Dam. A soaring structure that awes tourists as much today as it did when it was finished in 1936, the Hoover Dam was a gigantic project built in what was a remote, dusty, dangerous location. Yet it came in under budget and ahead of schedule. In the annals of big projects, it is a legend. In large part, that triumph was owed to Frank Crowe, the engineer who managed the project. Before tackling the Hoover Dam, Crowe had spent a long career building dams across the western United States and over those many years had developed a large and loyal team that followed him from project to project. The experience contained within that team was profound. So were the mutual trust, respect, and understanding.
The value of experienced teams cannot be overstated, yet it is routinely disregarded. A Canadian hydroelectric dam I consulted on is one of countless examples. It went ahead under the direction of executives who had zero experience with hydroelectric dams. Why? Because executives with experience were difficult to find. How hard can it be to deliver a big project?, the owners pondered. The oil and gas industry delivers big projects. A hydroelectric dam is a big project. Ergo, executives from oil and gas companies should be able to deliver a dam.
Or so the owners reasoned—and hired oil and gas executives to build the dam. The reader will not be surprised to learn that in sharp contrast to the Hoover Dam, this project turned into a fiasco that threatened the economy of a whole province.
The word deadline comes from the American Civil War, when prison camps set boundaries and any prisoner who crossed a line was shot.
Digital simulation enabled the second strategy: a radically different approach to construction. Instead of sending materials to a worksite to be measured, cut, shaped, and welded into buildings—the conventional way since the building of the pyramids—the materials were sent to factories, which used the detailed, precise digital specifications sent to them to manufacture components. Then the components were sent to the worksite to be assembled. To the untrained eye, T5 would have looked like a conventional construction site, but it wasn’t; it was an assembly site. The importance of this difference cannot be overstated, and every large construction site will need to follow suit if construction is going to make it into the twenty-first century.
By giving companies only positive incentives to perform well—including bonuses for meeting and beating benchmarks—it ensured that the interests of the many different companies working on the project were not pitted against one another.
There are five project types that are not fat-tailed. solar power, wind power, fossil thermal power (power plants that generate electricity by burning fossil fuels), electricity transmission, and roads. THIS MEANS THEY ARE TYPICALLY DELIVERED ON TIME AND BUDGET
The problem isn’t nuclear power; many other project types have track records only somewhat less bad.
Manufacturing in a factory and assembling on-site is far more efficient than traditional construction because a factory is a controlled environment designed to be as efficient, linear, and predictable as possible.
bad weather routinely wreaks havoc on outdoor construction, while production in a factory proceeds regardless of the elements.
Modularity can do astonishing things. When the Covid pandemic first emerged in China in January 2020, a company that makes modular housing modified an existing room design and cranked out units in a factory. Nine days later, a 1000-bed hospital with 1400 staff opened in Wuhan, ground zero of the outbreak.
So what sets the fortunate five apart? They are all modular to a considerable degree, some extremely so. Solar power? It’s born modular, with the solar cell as the basic building block. In a factory, put multiple solar cells together onto a panel. Ship and install the panel. Install another, and wire them together. Add another panel. And another until you have an array. Keep adding arrays until you are generating as much electricity as you like.
Wind power? Also extremely modular. Modern windmills consist of four basic factory-built elements assembled on-site: a base, a tower, the “head” (nacelle) that houses the generator, and the blades that spin. Snap them together, and you have one windmill. Repeat this process again and again, and you have a wind farm.
Fossil thermal power? Look inside a coal-burning power plant, say, and you’ll find that they’re pretty simple, consisting of a few basic factory-built elements assembled to make a big pot of water boil and run a turbine. They’re modular, much as a modern truck is modular. The same goes for oil- and gas-fired plants.
Electricity transmission? Parts made in a factory are assembled into a tower, and factory-made wires are strung along them. Repeat. Or manufactured cables are dug into the ground, section by section. Repeat again.
Roads? A multibillion-dollar freeway consists of several multimillion-dollar freeway sections strung together. Repeat, repeat, repeat.
At one extreme—the terrifying place where no one wants to be—we find storage of nuclear waste, hosting the Olympic Games, construction of nuclear power plants, building information technology systems, and constructing hydroelectric dams. They are all classic “one huge thing” projects.
Wind power must grow eleven-fold. Solar power must grow a mind-boggling twenty-fold.[37]
EMPIRE STATE BUILDING: WHY IT WAS A SUCCESS
the Empire State Building would be: slim, straight, and stretching higher into the sky than any other building on the planet.
In a 1931 publication, the corporation boasted that before any work had been done on the construction site “the architects knew exactly how many beams and of what lengths, even how many rivets and bolts would be needed. They knew how many windows Empire State would have, how many blocks of limestone, and of what shapes and sizes, how many tons of aluminum and stainless steel, tons of cement, tons of mortar. Even before it was begun, Empire State was finished entirely—on paper.
the Empire State Building was officially opened by President Herbert Hoover—exactly as scheduled,
The Empire State Building had been estimated to cost $50 million. It actually cost $41 million ($679 million in 2021). That’s 17% under budget. I call the pattern followed by the Empire State Building and other successful projects “Think slow, act fast.
another factor was his insistence that the project use existing, proven technologies “in order to avoid the uncertainty of innovative methods.” That included avoiding “hand work” whenever possible and replacing it with parts designed “so that they could be duplicated in tremendous quantity with almost perfect accuracy,” Lamb wrote, “and brought to the building and put together like an automobile on the assembly line.” Lamb minimized variety and complexity, including in floor designs, which were kept similar as much as possible. As a result, construction crews could learn by repeating. In other words, workers didn’t build one 102-story building, they built 102 one-story buildings.
the general contractors who erected the giant were Starrett Brothers and Eken, “a company with a proven track record of efficiency and speed in skyscraper construction,” noted the historian, Carol Willis.
it wasn’t the first time Lamb had designed the building. In Winston-Salem, North Carolina, the Reynolds Building, once the headquarters of the R. J. Reynolds Tobacco Company, is an elegant art deco structure that looks remarkably like a smaller, shorter Empire State Building.
DENMARK TRAIN TUNNEL UNDERWATER FAILURE
the government announced the Great Belt project. It comprised two bridges, one of which would be the world’s longest suspension bridge, to connect two of the bigger islands, including the one with Copenhagen on it. There would also be an underwater tunnel for trains—the second longest in Europe—which would be built by a Danish-led contractor. That was interesting because Danes had little experience boring tunnels. Things went wrong from the start. First there was a yearlong delay in delivering four giant tunnel-boring machines. Then, as soon as the machines were in the ground, they proved to be flawed and needed redesign, delaying work another five months. Finally, the big machines started slowly chewing their way under the ocean floor.
Up above, the bridge builders brought in a massive oceangoing dredger to prepare their worksite.[1] To do its work, the dredger stabilized itself by lowering giant support legs into the seafloor. When the work was done, the legs were lifted, leaving deep holes. By accident, one of the holes happened to be on the projected path of the tunnel. Neither the bridge builders nor the tunnelers saw the danger.
The machine and the whole tunnel flooded. So did a parallel tunnel and the boring machine within it.
the salt water in the tunnel was like acid to its metal and electronics. Engineers on the project told me at the time that it would be cheaper to abandon the tunnel and start again rather than pull out the borers, drain the tunnel, and repair it.
But politicians overrode them because an abandoned tunnel would be too embarrassing. Inevitably, the whole project came in very late and way over budget. The actual overrun was 55 percent, and 120 percent for the tunnel alone (in real terms, measured from the final investment decision