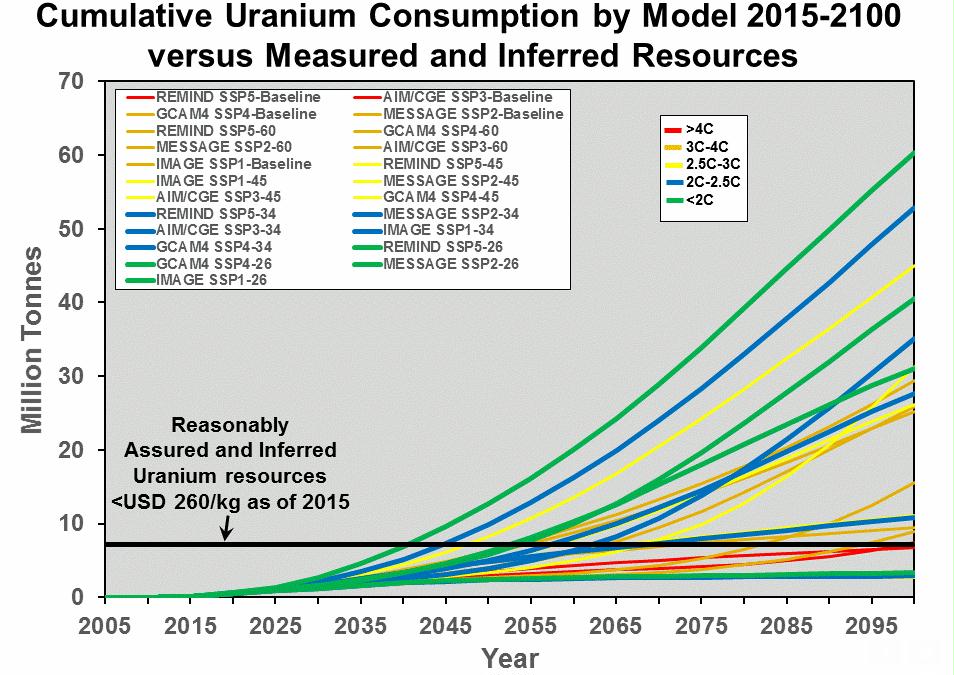
Figure 1. Cumulative uranium consumption by IPCC model 2015-2100 versus measured and inferred Uranium resources. Figure 1 shows that the next IPCC report counts very much on nuclear power to keep warming below 2.5 C. The black line represents how many million tonnes of reasonably and inferred resources under $260 per kg remain source: 2016 IAEA redbook Clearly most of the IPCC models are unrealistic. Source: David Hughes (private communication)
Preface. This is an extract of Ugo Bardi’s must read “Extracted” about the limits of production of uranium. You can find plenty of material saying there is are a lot of uranium reserves and resources left elsewhere (EMD 2019). The problem is, uranium requires fossil fuels to be mined, extracted, and processed, and world oil production peaked in 2018, peak world coal in 2013. If you read my book “When Trucks stop running”, you’ll see why trucks can’t run on electric batteries or overhead wires, and without trucks, civilization collapses, so nuclear electricity is not going to solve the energy crisis, and leaves toxic waste our descendants will have to deal with for hundreds of thousands of years (Alley 2013)
Uranium in the news:
Novikova T (2022) Russia & US Uranium. Counterpunch.org. The United States relies heavily on imported uranium, with Russia supplying about 16 percent. And also 23% of enrichment services are provided by Russia, so total imports may be more than 16%. Though so many reactors in the U.S. are long past their time of retirement and are shutting down that this may well reduce consumption by 16% or more. The U.S. only produced 1% of world uranium production, and new permits take years.
July 2016 Water power. Extracting uranium from seawater. Scientific American. Stephen Kung of the DOE’s office of Nuclear Energy said that terrestrial sources of uranium are expected to last for only another 100 to 200 more years. It takes 8 weeks to extract 6 grams of uranium from seawater, or 0.75 grams per day, It takes 27,000,000 grams to run a 1 gigawatt nuclear power plant for one year, so it would take 98,630 years to extract enough uranium from seawater to run just one nuclear power plant.
Alice Friedemann www.energyskeptic.com Author of Life After Fossil Fuels: A Reality Check on Alternative Energy; When Trucks Stop Running: Energy and the Future of Transportation”, Barriers to Making Algal Biofuels, & “Crunch! Whole Grain Artisan Chips and Crackers”. Women in ecology Podcasts: WGBH, Planet: Critical, Crazy Town, Collapse Chronicles, Derrick Jensen, Practical Prepping, Kunstler 253 &278, Peak Prosperity, Index of best energyskeptic posts
***
Bardi, Ugo. 2014. Extracted: How the Quest for Mineral Wealth Is Plundering the Planet. Chelsea Green Publishing.
Although there is a rebirth of interest in nuclear energy, there is still a basic problem: uranium is a mineral resource that exists in finite amounts.
Even as early as the 1950s it was clear that the known uranium resources were not sufficient to fuel the “atomic age” for a period longer than a few decades.
That gave rise to the idea of “breeding” fissile plutonium fuel from the more abundant, non-fissile isotope 238 of uranium. It was a very ambitious idea: fuel the industrial system with an element that doesn’t exist in measurable amounts on Earth but would be created by humans expressly for their own purposes. The concept gave rise to dreams of a plutonium-based economy. This ambitious plan was never really put into practice, though, at least not in the form that was envisioned in the 1950s and ’60s. Several attempts were made to build breeder reactors in the 1970s, but the technology was found to be expensive, difficult to manage, and prone to failure. Besides, it posed unsolvable strategic problems in terms of the proliferation of fissile materials that could be used to build atomic weapons. The idea was thoroughly abandoned in the 1970s, when the US Senate enacted a law that forbade the reprocessing of spent nuclear fuel.
A similar fate was encountered by another idea that involved “breeding” a nuclear fuel from a naturally existing element—thorium. The concept involved transforming the 232 isotope of thorium into the fissile 233 isotope of uranium, which then could be used as fuel for a nuclear reactor (or for nuclear warheads). 48 The idea was discussed at length during the heydays of the nuclear industry, and it is still discussed today; but so far, nothing has come out of it and the nuclear industry is still based on mineral uranium as fuel.
Today, the production of uranium from mines is insufficient to fuel the existing nuclear reactors. The gap between supply and demand for mineral uranium has been as large as almost 50% from 1995 to 2005, though gradually reduced the past few years.
The U.S. mined 370,000 metric tons the past 50 years, peaking in 1981 at 17,000 tons/year. Europe peaked in the 1990s after extracting 460,000 tons. Today nearly all of the 21,000 ton/year needed to keep European nuclear plants operating is imported.
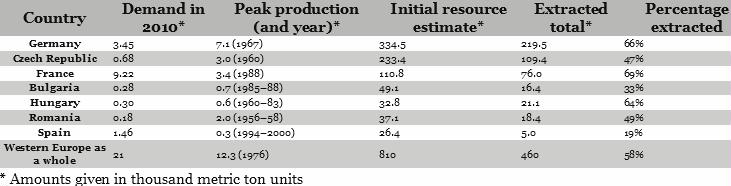
Table 1. The European mining cycle allows us to determine how much of the originally estimated uranium reserves could be extracted versus what actually happened before it cost too much to continue. Remarkably in all countries where mining has stopped it did so at well below initial estimates (50 to 70%). Therefore it’s likely ultimate production in South Africa and the United States can be predicted as well.
The Soviet Union and Canada each mined 450,000 tons. By 2010 global cumulative production was 2.5 million tons. Of this, 2 million tons has been used, and the military had most of the remaining half a million tons.
The most recent data available show that mineral uranium accounts now for about 80% of the demand. The gap is filled by uranium recovered from the stockpiles of the military industry and from the dismantling of old nuclear warheads.
This turning of swords into plows is surely a good idea, but old nuclear weapons and military stocks are a finite resource and cannot be seen as a definitive solution to the problem of insufficient supply. With the present stasis in uranium demand, it is possible that the production gap will be closed in a decade or so by increased mineral production. However, prospects are uncertain, as explained in “The End of Cheap Uranium.” In particular, if nuclear energy were to see a worldwide expansion, it is hard to see how mineral production could satisfy the increasing uranium demand, given the gigantic investments that would be needed, which are unlikely to be possible in the present economically challenging times.
At the same time, the effects of the 2011 incident at the Fukushima nuclear power plant are likely to negatively affect the prospects of growth for nuclear energy production, and with the concomitant reduced demand for uranium, the surviving reactors may have sufficient fuel to remain in operation for several decades.
It’s true that there are large quantities of uranium in the Earth’s crust, but there are limited numbers of deposits that are concentrated enough to be profitably mined. If we tried to extract those less concentrated deposits, the mining process would require far more energy than the mined uranium could ultimately produced [negative EROI].
Modeling Future Uranium Supplies
Michael Dittmar used historical data for countries and single mines, to create a model that projected how much uranium will likely be extracted from existing reserves in the years to come. The model is purely empirical and is based on the assumption that mining companies, when planning the extraction profile of a deposit, project their operations to coincide with the average lifetime of the expensive equipment and infrastructure it takes to mine uranium—about a decade.
Gradually the extraction becomes more expensive as some equipment has to be replaced and the least costly resources are mined. As a consequence, both extraction and profits decline. Eventually the company stops exploiting the deposit and the mine closes. The model depends on both geological and economic constraints, but the fact that it has turned out to be valid for so many past cases shows that it is a good approximation of reality.
This said, the model assumes the following points:
- Mine operators plan to operate the mine at a nearly constant production level on the basis of detailed geological studies and to manage extraction so that the plateau can be sustained for approximately 10 years.
- The total amount of extractable uranium is approximately the achieved (or planned) annual plateau value multiplied by 10.
Applying this model to well-documented mines in Canada and Australia, we arrive at amazingly correct results. For instance, in one case, the model predicted a total production of 319 ± 24 kilotons, which was very close to the 310 kilotons actually produced. So we can be reasonably confident that it can be applied to today’s larger currently operating and planned uranium mines. Considering that the achieved plateau production from past operations was usually smaller than the one planned, this model probably overestimates the future production.
Table 2 summarizes the model’s predictions for future uranium production, comparing those findings against forecasts from other groups and against two different potential future nuclear scenarios.
As you can see, the forecasts obtained by this model indicate substantial supply constraints in the coming decades—a considerably different picture from that presented by the other models, which predict larger supplies.
The WNA’s 2009 forecast differs from our model mainly by assuming that existing and future mines will have a lifetime of at least 20 years. As a result, the WNA predicts a production peak of 85 kilotons/year around the year 2025, about 10 years later than in the present model, followed by a steep decline to about 70 kilotons/year in 2030. Despite being relatively optimistic, the forecast by the WNA shows that the uranium production in 2030 would not be higher than it is now. In any case, the long deposit lifetime in the WNA model is inconsistent with the data from past uranium mines. The 2006 estimate from the EWG was based on the Red Book 2005 RAR (reasonably assured resources) and IR (inferred resources) numbers. The EWG calculated an upper production limit based on the assumption that extraction can be increased according to demand until half of the RAR or at most half of the sum of the RAR and IR resources are used. That led the group to estimate a production peak around the year 2025.
Assuming all planned uranium mines are opened, annual mining will increase from 54,000 tons/year to a maximum of 58 (+ or – 4) thousand tons/year in 2015. [ Bardi wrote this before 2013 and 2014 figures were known. 2013 was 59,673 (highest total) and 56,252 in 2014.]
Declining uranium production will make it impossible to obtain a significant increase in electrical power from nuclear plants in the coming decades.
Here are 7 other posts from this great book:
- Mining: Waste, Pollution, Destruction
- Ugo Bardi predictions of the future
- Minerals and War
- Minerals: Natural gas
- Minerals: Coal
- Mineral: Soil
- Peak Uranium
References
Alley, W. M., et al. 2014. Too Hot to Touch: The Problem of High-Level Nuclear Waste.Cambridge University Press.
EMD. 2019. EMD Uranium (Nuclear minerals and REE) committee annual report. i2massociates.com
5 Responses to Peak Uranium by Ugo Bardi from Extracted: How the Quest for Mineral Wealth Is Plundering the Planet