Preface. Today the electric grid stays up because of months of backup power from natural gas, coal, and uranium. Most of all natural gas because it can quickly balance wind and solar as they suddenly appear or die out. Natural gas also provides peak power during the hottest and coldest times of the year, and ramps up as the sun goes down massively, since this happens before peak evening demand. Geothermal power can’t provide this balance, it can only be used like coal and nuclear to provide steady baseload power. Still, wind and solar need all the help they can get, especially in California where all of the best wind and solar sites have been built out.
How geothermal works and where located: A Visual Crash Course on Geothermal Energy
Alice Friedemann www.energyskeptic.com Author of Life After Fossil Fuels: A Reality Check on Alternative Energy; When Trucks Stop Running: Energy and the Future of Transportation”, Barriers to Making Algal Biofuels, & “Crunch! Whole Grain Artisan Chips and Crackers”. Women in ecology Podcasts: WGBH, Planet: Critical, Crazy Town, Collapse Chronicles, Derrick Jensen, Practical Prepping, Kunstler 253 &278, Peak Prosperity, Index of best energyskeptic posts
***
Who cares about geothermal? Drill anywhere on earth
The idea is that rather than limiting geothermal to the very few natural hot water geothermal reservoirs near the surface, go anywhere in the world by drilling deep enough to reach hot rocks.
One reason this idea is gaining ground is the advanced drilling techniques developed by the oil and gas industry to go after shale “fracked” hydrocarbons.
But geothermal drilling is different and far more expensive. The harder the rock, the more time and energy it takes to bore through. The sedimentary rocks that oil and gas companies get hydrocarbons from are much softer than the rocks drilled through for geothermal wells. The hole diameter for geothermal is also more than twice the size of oil and gas wells, requiring even more casing and cement in construction.
The deeper the well, the higher the temperature, so both dry heat and geothermal well drill bits and pipes need expensive alloys with significant amounts of chromium, manganese, cobalt, and titanium to cope.
But you can’t just drill anywhere. The location needs to be where fluid exists to transport heat to the surface, and enough water for turbines to generate steam heat to make electricity.
Water is also needed to cool the drilling equipment to make it last longer.
Nor can it be done economically in granite and many other types of hard rock.
The pipes are very expensive no matter where drilling takes place, because a deep well might have a million pounds of piping. They need to be thick and heavy to survive pumping pressures of 40-50 pounds per foot. The sheer amount of weight can break the pipe if it isn’t high quality, and eventually it is hard to find the hoisting equipment with enough power to lift it.
Despite doing all of this well so far, if the rocks aren’t stable, the hole may collapse, or blowout from pressurized fluids flowing up from the deep. Then there are deep rocks that leach toxic and radioactive materials, increasing the cost of disposal and hazardous to touch.
If the search for heat goes below 4900 feet (1500 meters) deep, the earthquake potential increases (Minetto 2020).
An annoying amount of rock from the hole needs to be removed which will get harder and harder as drilling deepens.
Drilling is hard and expensive, finding a site even harder
The harder the rock, the more energy it takes to crush and time to drill through. The sedimentary rocks that oil and gas companies drill through are softer than the rocks drilled through for geothermal wells. The hole diameter is also more than twice the size of oil and gas wells, requiring more casing and cement in construction. Plus due to the harsh environment, geothermal wells need more expensive alloys containing significant amounts of expensive chromium, manganese, cobalt, and titanium to cope with the high temperatures. Commercial facilities are all at locations where fluid exists to transport heat to the surface. Such sites are very limited, all of them in the western united states. They are very hard to find too, needles in a haystack (Young 2019).
Geothermal provides less than half a percent of U.S. electric power
NREL (2016) recently looked at whether the current 3.8 GWe could be doubled to 7.6 GWe by 2020. Only 0.784 GWe was likely, with another 0.856 GWe possible with expedited development (these projects often take 5 years), and another 1.722 GWe if financing could be found and permits given. The report concluded it was unlikely that doubling geothermal electricity capacity could happen by 2020.
There’s not enough geothermal to make a dent (Murphy 2012).
Abundant, potent, or niche? Hmmm. It’s complex. On paper, we have just seen that the Earth’s crust contains abundant thermal energy, with a very long depletion time. But extraction requires a constant effort to drill new holes and share the derived heat. Globally we use 12 TW of energy. Heat released from all land is 9 TW, but practical utilization is impossible. For one thing, the efficiency with which we can produce electricity dramatically reduces the cap to the 2 TW scale. And for heating just 1 home, you’d need to capture heat from an area 100 meters on a side. Clearly, geothermal energy works well in select locations (geological hotspots). But it’s too puny to provide a significant share of our electricity, and direct thermal use requires substantial underground volumes/areas to mitigate depletion. All this on top of requirements to place lots of tubing infrastructure kilometers deep in the rock (do I hear EROEI whimpering?). And geothermal is certainly not riding to the rescue of the imminent liquid fuels crunch.
Geothermal can’t balance wind & solar to keep the grid up
Geothermal can’t be quickly ramped up and down to balance intermittent, variable, unreliable wind and solar power either, and the grid must be kept within a very narrow range to prevent blackouts.
Geothermal sites can drive species extinct and damage sites sacred to Native Americans
The endangered Dixie Valley toad and a lawsuit from a nearby Native American tribe (it would destroy sacred hot springs) are preventing a geothermal plant from being built in the Nevada desert. The Center for Biological Diversity points out that in the Great Basin, hot springs and thermal water features are oases of biodiversity, providing water in the driest place in North America and thermal refuge in the coldest desert in North America (Chung 2022).
Geothermal consumes massive amounts of water & energy
Geothermal plants use a lot of energy to keep the water hot enough to prevent minerals from precipitating out and clogging pipes and heat exchangers. Some were poorly managed and exhausted after running out of water. Maintenance costs are high due to corrosion from corrosive gases and aerosols, etc.
“As with fossil fuel power plants and concentrating solar power, increases in air and water temperatures can reduce the efficiency with which geothermal facilities generate electricity, according to DOE’s 2013 assessment. Geothermal power plants can also withdraw and consume significant quantities of water, according to DOE, making them susceptible to water shortages caused by changes in precipitation or warming temperatures” (USGAO 2014).
94% of all known U.S. geothermal resources are located in California.
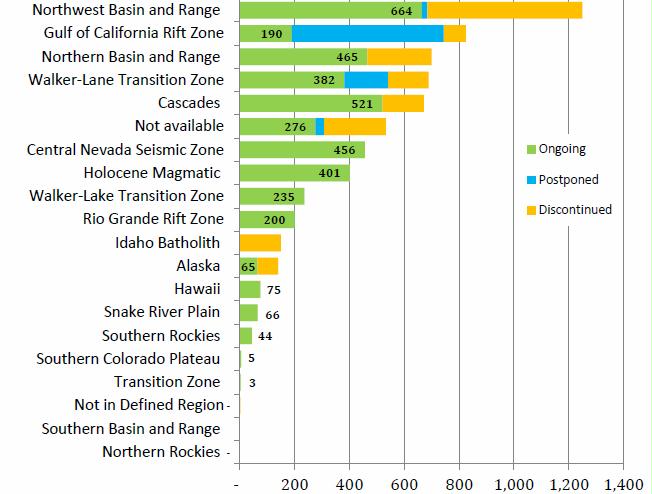
Figure 2. Source: NREL 2016. U.S. geothermal total estimated project capacity (in megawatts) by geothermal region. This figure highlights areas that had significant proportions of projects that were either discontinued or postponed: Idaho Batholith (100%), Gulf of California Rift Zone (77%), Alaska (45%), Northwest Basin and Range (47%), the Walker-Lane Transition Zone (44%), and the Northern Basin and Range (36%).
Lack of transmission lines strands many geothermal sites
Only a few urban areas in California and other states with geothermal resources (i.e. volcanoes, hot springs, and geysers) are near enough to exploit them. This is because the cost of adding very long transmission lines to faraway geysers can make a geothermal resource too expensive. Even sites closer to cities are very expensive to build. On top of that, unless the geothermal resource is very large, more of the power is lost over transmission lines than conventional power plants (CEC 2014 page 73).
The largest and most potentially lucrative markets are generally far from the best geothermal resource areas. New lines could bring these resources to market, but the economics of building new transmission are extremely problematic for small geothermal plants. A long-distance line sized to a handful of small projects is costly and loses a great deal of power along the way; a high-capacity line brings greater efficiency, but only if the capacity connecting to it is enough to keep the utilization rate high. These complications offset geothermal’s comparative advantage with respect to providing base load power. Billions of dollars are at stake for any major long-distance transmission project, and completion can take seven years or longer. So unless the geothermal plants have already been built a utility is unlikely to build the lines to distant geothermal sites, which in turn can’t build them without transmission lines (NREL 2012, Black 2010, BPA 2016, Driesen 2006, GEA 2007, Hurlbut 2010, MIT 2006, NAERC 2009, NREL 2010, Pletka 2009, RMOTC 2010, Senate hearing 2006, DOE 2010, WECC 2010, WGA 2009).
Getting the financing is hard
The current environment for financing independent power projects is challenging. These challenges include weak corporate profits, changes in corporate direction, and heightened risk aversion. As a result, a number of the financial institutions that were lead underwriters in the past are either pulling out of the market or are taking a lower profile in project financing.
Lenders don’t like geothermal projects because they have very high upfront costs that take over 5 years for returns to start coming in. Or never come in, the rock temperature and permeability three kilometers below is not predictable (Slav 2020).
Biomass and geothermal projects are considered riskier than natural gas, solar, and wind projects. This is seen in the lower leverage, higher pricing, and higher DSCRs than for the other generating technologies. The higher level of project risk for biomass and geothermal projects is partly attributed to the technology and fuel sources. Solid fuel power plants require more project infrastructure than do other fuel types. Geothermal projects have inherently uncertain steam supplies as has been seen at the Geysers. Some of the risk also is based on the relatively small number of these projects being developed.
The steadily increasing wheeling access charges the California ISO expects to put in place over the next decade represent a growing, significant cost to renewable developers who find their best renewable resources in locations that are distant from demand.
They can be risky to develop since they don’t always work out. In June 1980, Southern California Edison (SCE) began operation of a 10 MW experimental power plant at the Brawley geothermal field, also in Imperial County. However, after a few years of operation further development was ceased due to corrosion, reservoir uncertainties, and the presence of high salinity brines.
Geothermal groups reported that most attempts to develop geothermal resources for electricity generation are unsuccessful, that costs to develop geothermal power plants can surpass $100 million, and that it can take 3 to 5 years for plants to first produce and sell electricity. Although some geothermal resources are easy to find because they produce tell-tale signs such as hot springs, most resources are buried deep within the earth—at depths sometimes exceeding 10,000 feet—and finding them often requires an in-depth knowledge of the area’s geology, geophysical surveys, remote sensing techniques, and at least one test well. The risks and high initial costs associated with exploring for and developing geothermal resources limit financing. Moreover, few lenders will finance a geothermal project until a contract has been signed by a utility or energy marketer to purchase the anticipated electricity. Geothermal industry officials describe the process of securing a contract to sell electricity as complicated and costly (Senate Hearing 2006).
Geothermal can be seasonal
They often generate less power in the summer (NREL 2016).
Issues with geothermal installations
There are two components to the geothermal resource base: hydrothermal (water heated by Earth) that exists down to a depth of about 3 km, and enhanced geothermal systems (EGS) associated with low-permeability or low-porosity heated rocks at depths down to 10 km.
A National Academy of Sciences concluded that hydrothermal resources are too small to have a major overall impact on total electricity generation in the United States — at best 13 GW of electric power capacity in identified resources (NAS 2009).
The largest geothermal installation in the world is the Geysers in Northern California, occupying 30 square miles. The 15 power plants have a total net generating capacity of about 725 MW of electricity—enough to power 725,000 homes (Heinberg).
- Geothermal plants often emit hydrogen sulfide, CO2, and toxic sludge containing arsenic, mercury, sulfur, and silica compounds.
- Extra land may be needed to dispose of wastes and excess salts
- groundwater and freshwater can be a limiting force since both hydrothermal and dry rock systems need water
- Maintenance costs are high because the steam is corrosive and deposits minerals, which clogs pipes and destroys valves.
- When you extract energy from just about anything it decreases, the same is true for geothermal. So you endlessly need to keep looking for more prospects. For example, the “Geysers” area of Northern California has gone from 2000 MWe to 850 MWe since it was first tapped for power. J. Coleman. 15 Apr 20001. Running out of steam: Geothermal field tapped out as alternative energy source. Associated Press.
- We need a breakthrough in materials that won’t melt to drill deeply enough to get significant power in non-geothermal areas.
- You can lose a significant amount of steam because the water you pour down the hole is so hot it fractures rocks and escapes into cracks before it can return up the steam vent. Over time, less and less steam for power generation is produced.
- If you wanted to tap the heat without any geothermal activity, it becomes energy intensive, because you have to drill much deeper (geothermal sources are already near the surface), the rock below has to be fractured (which it already is in geothermal regions) to release steam, and fracturing and keeping the rock fractured takes far more ongoing energy than the initial drilling.
- No one has figured out how to do hot dry rock economically – time’s running out.
- Even if Geodynamics succeeds in scaling their experiments into a real geothermal power plant, it will in huge part be due to the location: “This is the best spot in the world, a geological freak,” Geodynamics managing director Bertus de Graaf told Reuters. “It’s really quite serendipitous, the way the elements — temperature, tectonics, insulating rocks — have come together here.
Although it would be great if we could access the heat 3 to 10 km below the earth, such operations would cool down so much that they’d have to be shut down within 20 to 30 years, and production wells would need to be re-drilled every 4 to 8 years meanwhile. We don’t know how to do this anyhow. Despite oil and gas drilling, we don’t have much experience going this deep or know how to enhance heat transfer performance for lower-temperature fluids in power production. Another challenge is to improve reservoir-stimulation techniques so that sufficient connectivity within the fractured rock can be achieved. France has been trying to make this work for over 2 decades, so don’t hold your breath (NAS 2009).
Geothermal Technology Costs
Geothermal technologies remain viable in California, although they are subject to a number of limitations that are likely to reduce the number of sites developed in California.
Geothermal resource costs are driven largely by the highly variable and significant costs of drilling and well development. These costs are unique to each site and represent a significant risk on the part of the developer. While a successful well may be able to produce electricity at low cost, other wells in the same area may require much more investment in time and resources before they are producing efficiently. Costs for new geothermal plants are projected to increase slightly over the coming years. Limitations of location and drilling are unlikely to see improvement in California, while nationally there are very few geothermal projects under development.
Factors Affecting Future Geothermal Development
California’s relative abundance of geothermal resources in comparison to the rest of the United States does not mean that geothermal power production would be viable or cost-effective everywhere in the state. Developers must consider multiple factors of cost and viability when deciding where to locate new geothermal plants. In turn, these considerations drive the estimates of future costs of new geothermal power plants in California. Considerations for developing geothermal power plants in liquid-dominated resources include (Kagel, 2006):
- Exploration Costs- Exploration and mapping of the potential geothermal resource is a critical and sometimes costly activity. It effectively defines the characteristics of the geothermal resource.
- Confirmation Costs-These are costs associated with confirming the energy potential of a resource by drilling production wells and testing their flow rates until about 25% of the resource capacity needed by the project is confirmed.
- Site/Development Costs- Covering all remaining activities that bring a power plant on line, including: Drilling- The success rate for drilling production wells during site development average 70 to 80% (Entingh et al 2012). The size of the well and the depth to the geothermal reservoir are the most important factors in determining the drilling cost.
- Project leasing and permitting-Like all power projects, geothermal plants must comply with a series of legislated requirements related to environmental concerns and construction criteria.
- Piping network- The network of pipes are needed to connect the power plant with production and injection wells. Production wells bring the geothermal fluid (or brine) to the surface to be used for power generation, while injection wells return the used fluid back to the geothermal system to be used again.
- Power plant design and construction- In designing a power plant, developers must balance size and technology of plant materials with efficiency and cost effectiveness. The power plant design and construction depends on type of plant (binary or flash) as well as the type of cooling cycle used (water or air cooling).
- Transmission- Includes the costs of constructing new lines, upgrades to existing lines, or new transformers and substations.
Another important factor contributing to overall costs is O&M costs, which consist of all costs incurred during the operational phase of the power plant (Hance 2005). Operation costs consist of labor; spending for consumable goods, taxes, royalties; and other miscellaneous charges.
Maintenance costs consist of keeping equipment in good working status. In addition, maintaining the steam field, including servicing the production and injection wells (pipelines, roads, and so forth) and make-up well drilling, involves considerable expense.
Development factors are not constant for every geothermal site. Each of the above factors can vary significantly based on specific site characteristics.
Make-up drilling aims to compensate for the natural productivity decline of the project start-up wells by drilling additional production wells. drive costs for geothermal plants (not mentioned directly above since they are highly project specific) are project delays, temperature of the resource, and plant size.
The temperature of the resource is an essential parameter influencing the cost of the power plant equipment. Each power plant is designed to optimize the use of the heat supplied by the geothermal fluid. The size, and thus cost, of various components (for example, heat exchangers) is determined by the temperature of the resource. As the temperature of the resource increases, the efficiency of the power system increases, and the specific cost of equipment decreases as more energy is produced with similar equipment. Since binary systems use lower resource operating temperatures than flash steam systems, binary costs can be expected to be higher. Figure 33 provides estimates for cost variance due to resource temperature. As the figure shows, binary systems range in cost from $2,000/kWh to slightly more than $4,000/kWh, while flash steam systems range from $1,000/kWh to just above $3,000/kWh (Hance, 2005).
Technology Development Considerations
In addition to the cost factors listed in the previous section of the report addressing geothermal binary plants, for some flash plants a corrosive geothermal fluid may require the use of resistive pipes and cement. Adding a titanium liner to protect the casing may significantly increase the cost of the well. This kind of requirement is rare in the United States, found only in the Salton Sea resource in Southern California (Hance, 2005).
What about using abandoned oil wells to generate thermal power?
Practically, it’s not that straightforward. First, flow rates from such wells are much lower than from newly drilled geothermal wells. Second, there is the issue of heat: most oil wells are simply not hot enough to make economic sense if we are talking about electricity generation. Third, they’re not likely to last long, not even 20-40 years since most existing wells suffer from well integrity issues. Rather than generating electricity these oil wells could be used to generate heat, but that requires constant refreshment with water, and it it takes a long time for the water to heat up enough, this isn’t a viable option. And it is expensive to inject water and construct heat transferring equipment. Most old wells are far from towns and cities, so it would be expensive to pump water up from far below into a pipeline with hot water to heat dwellings. Filling up old coal mines with water to heat it or generate electricity runs into the same issues (Slav 2021).
Many geothermal resources are hot enough
Only the highest temperature geothermal resources, generally above 200 degrees Fahrenheit, are suitable for electricity generation (Senate Hearing 2006).
Enhanced Geothermal has a long way to go (Selvans 2013, Trainer 2012, Ziagos 2013, DOE 2008)
Clearly if we could drill down into the earth anywhere and pour water down the hole for steam turbines, there would be an enormous amount of energy that could be generated. This is what is meant by “enhanced” geothermal systems (EGS).
The drilling process to do this is very similar to fracking, but far more advanced techniques need to be developed for EGS. The deeper the drill bit goes, the more expensive it gets, and the more likely it is to be destroyed from high temperatures or worn down.
Attempts to drill 2 km down or more have been going on since the early 1970s. But the same problems that occurred back then are still occurring now:
- Huge water losses (70 to 90% of the water disappears).
- Permeability issues. Minerals such as silica and lithium precipitate through flow channels, reducing fracture permeability. Removing the minerals is an expensive, time-consuming process that limits the usefulness of enhanced geothermal recovery.
- Short-circuiting between the injection and production wells
- mechanical failures,
- Insufficient connectivity between wells to meet economic requirements for reservoir productivity over its lifetime, etc.
- Some of the few demonstration projects are “cheating” in that they’re on the edges of existing hydrothermal systems.
- “Lessons learned” may not apply to new wells since the geology of each area is unique
- Most of the areas under consideration are the Basin and Range faultlines, where there is very little water to pour down drilled holes.
- In most areas of the country the depths to drill down to, or the kinds of rocks to drill through, or a lack of huge amounts of water to pour down the hole can rule out many areas.
- Lack of models to improve drilling techniques by simulating the movement of fluid through rocks at critical and super-critical conditions
- Objections to these attempts by nearby residents include worries about earthquakes and the chemicals being injected.
The depth to be drilled down to is so deep that it is likely this technology will always be too expensive and use more energy to drill than obtained. The EROI of EGS is hard to even guess at since there are no commercial plants, so it’s unknown how (un)productive an imaginary EGS will be. It depends on an unknown reservoir depth, unknown temperature, an unknown temperature decline rate, an unknown amount of energy to correct impermeability issues, an unknown amount of energy to move water from the nearest abundant source to pour it into the bowels of the earth, and so on.
REFERENCES
Black (2010) Utah Renewable Energy Zone Phase 2 Final Report.
BPA (2016) 2006 Pacific Northwest Loads & Resources Study. Bonneville Power Administration
Bradley, Robert L., Jr. Geothermal: The Nonrenewable Renewable. National Center for Policy Analysis.
CEC (2014) Estimated cost of new renewable and fossil generation in California. California Energy Commission.
Chung C (2022) With U.S. Protection, a Tiny Toad Could Upend a Geothermal Project. New York Times.
DOE (2008) Geothermal Tomorrow. US Department of Energy.
DOE (2010) (September 2010). “Low-Temperature, Coproduced, and Geopressured Geothermal Technology Strategic Action Plan. U.S. Department of Energy.
Driesen J (2006) Distributed Generation: Challenges and Possible Solutions. 2006 IEEE Power Engineering Society General Meeting, Montreal, Canada, Federal Energy Regulatory Commission, 18 CFR Parts 35 and 37 (Order 890).
GEA (2007, 2008) The State of Geothermal Technologies,” Part 1 (2007) and Part 2 (2008). Geothermal Energy Association.
Hance C (2005) Factors Affecting Costs of Geothermal Power Development, Geothermal Energy Association.
Heinberg R (2009) Searching for a Miracle. “Net Energy” Limits & the Fate of Industrial Society. Post Carbon Institute.
Hurlbut DJ (2010). Multistate Decision Making for Renewable Energy and Transmission: An Overview. University of Colorado Law Review 81: 677-703.
Kagel, A. October 2006. A Handbook on the Externalities, Employment, and Economics of Geothermal Energy. Geothermal Energy Association.
Minetto R et al (2020) Tectonic and Anthropogenic Microseismic Activity While Drilling Toward Supercritical Conditions in the Larderello-Travale Geothermal Field, Italy. Journal of Geophysical Research: Solid Earth
MIT (2006) (2006) The Future of Geothermal Energy: Impact of Enhanced Geothermal Systems (EGS) on the United States in the 21st Century. Massachusetts Institute of Technology.
Murphy T (2012) Warm and Fuzzy on Geothermal? Do the Math.
NAERC (2009) Accommodating High Levels of Variable Generation,” special report. North American Electric Reliability Corporation
NAS (2009) America’s Energy Future: Technology and Transformation. 2009. National Academy of Sciences, National Research Council, National Academy of Engineering.
NREL (2010) Western Wind and Solar Integration Study. Report No. SR-550-47434. Golden, CO: National Renewable Energy Laboratory
NREL (2012) Geothermal power and interconnection. The Economics of Getting to Market. National Renewable Energy Laboratory. Technical Report NREL/TP-6A20-54192
NREL (2016) Doubling Geothermal Generation Capacity by 2020: A Strategic Analysis. National Renewable Energy Laboratory. Technical Report NREL/TP-6A20-64925
Oberhaus D (2020) Want Unlimited Clean Energy? Just Drill the World’s Hottest Well. Wired.com
Pletka R (2009). Western Renewable Energy Zones, Phase 1: QRA Identification Technical Report. NREL/SR-6A2-46877. Golden, CO: National Renewable Energy Laboratory.
RMOTC (2010) ld Testing Center. (March 2010). Ormat: Low-Temperature Geothermal Power Production. http://www.rmotc.doe.gov/PDFs/Ormat_report.pdf.
Selvans Z (2013) Enhanced Geothermal Systems promise dispatchable zero carbon power. cleanenergyaction.org
Senate hearing (2006) Implementation of the provisions of the energy policy act of 2005. Enhancing oil and gas production; geothermal energy and other renewables; and hydrogen and fuel cell research and development. U.S. Senate hearing 109-503. Testimony of Jim Wells, Director, Natural Resources & Environment, Government Accountability Office
Slav I (2020) What’s Holding Geothermal Energy Back? oilprice.com
Slav I (2021) Can Abandoned Oil Wells Be Used To Generate Geothermal Power? oilprice.com
Trainer T (2012) A critique of Jacobson and Delucchi’s proposals for a world renewable energy supply. Energy Policy 44, 476–481.
USGAO (2014) Climate Change: Energy Infrastructure Risks and Adaptation Efforts GAO-14-74. United States Government Accountability Office.
WECC (2010) 2010 Western Interconnection Transmission Path Utilization Study: Path Flows, Schedules and OASIS ATC Offerings. Western Electric Coordinating Council.
WGA (2009) Western Renewable Energy Zones: Phase 1 Report. Western Governors’ Association
Young KR (2019) Opportunities and challenges for advanced geothermal energy development in the US. US Senate hearing June 20.
Ziagos J (2013) A technology roadmap for strategic development of enhanced geothermal systems. proceedings of the 38th workshop on geothermal reservoir engineering, Stanford University, CA.